Anaerobic Soil Disinfestation for Management of Soilborne Pests in Vegetables
ID
SPES-326NP
Introduction
Soilborne pathogens are a constant threat to vegetable crops across Virginia. Fumigants and fungicides are the most common methods used to manage soilborne pathogens in bedding vegetables; however, the phase-out of methyl bromide and the high cost and toxicity of other available fumigants have created a need for additional economic and environmentally friendly options to treat soilborne plant pathogens. Anaerobic soil disinfestation (ASD) is a technique that was developed independently in Japan and the Netherlands in the early 2000’s to manage soilborne diseases.
How it Works
Anaerobic soil disinfestation is achieved by incorporating carbon source amendments into the soil, covering with a standard polyethylene mulch (used in commercial plasticulture production systems) and irrigating to field capacity over a 2- to 4-week period. The process creates anaerobic conditions that are detrimental to most soilborne pathogens. The soil is a habitat for a vast community of microbes, some of which depend exclusively on oxygen and others of which can survive in anaerobic conditions. ASD shifts soil microbe populations from aerobic to facultatively and obligately anaerobic organisms. The subsequent production of toxic products, such as organic acids and volatile compounds, along with low oxygen levels, contribute to suppression of soilborne pathogens which cannot persist under these conditions.
ASD Efficacy
Various studies throughout the U.S. have shown that ASD is a useful technique to suppress some soilborne plant pathogens, such as the fungal pathogens, Sclerotium rolfsii, Fusarium oxysporum, Verticillium dahliae, and Rhizoctonia solani; the oomycete, Pythium ultimum; the bacterium, Ralstonia solanacearum; plant-parasitic nematodes, Meloidogyne incognita (root-knot nematode) and Globodera pallida (potato cyst nematode), and certain weeds (e.g. Amaranthus retroflexus, Chenopodium album, and Cyperus esculentus) (Strauss and Kluepfel 2015; Shrestha et al. 2016). The efficacy of ASD depends largely on factors such as, environmental conditions, treatment length, soil temperatures, and carbon sources. Some experiments have achieved up to 91% suppression of fungal growth with ASD, while less suppression has been observed with weeds (82%) and nematodes (20-40%) (Shrestha et al. 2016). In Virginia, ongoing studies are assessing different carbon sources (dry and cover crop biomass) and rates to use with ASD in the management of soilborne pathogens and foodborne pathogens.
Carbon Sources and Rates
The rapid growth of microorganisms in the soil depends directly on the available carbon; therefore, the carbon source amendment directly influences the effect of ASD on soil microbes. Studies have evaluated the use of dry and blackstrap molasses (8 tons/A), wheat bran (4-8 tons/A), rice bran (4-8 tons/A), ethanol (2-10% v/v), and cover crops, such as cowpea (Vigna unguiculata), sunn hemp (Crotalaria juncea), sorghum-sudangrass (Sorghum bicolor Moench x S. bicolor var. Sudanese), and pearl millet (Pennisetum glaucum) (9 to 27 lb of dry weight per acre, depending on cover crop), with promising results in managing some soilborne diseases (Strauss and Kluepfel 2015). In VA, ongoing studies are assessing the effectiveness of locally available carbon sources and rates (Fig. 1) for use in ASD, as well as the effectiveness of cover crop biomass on inducing anaerobic conditions.
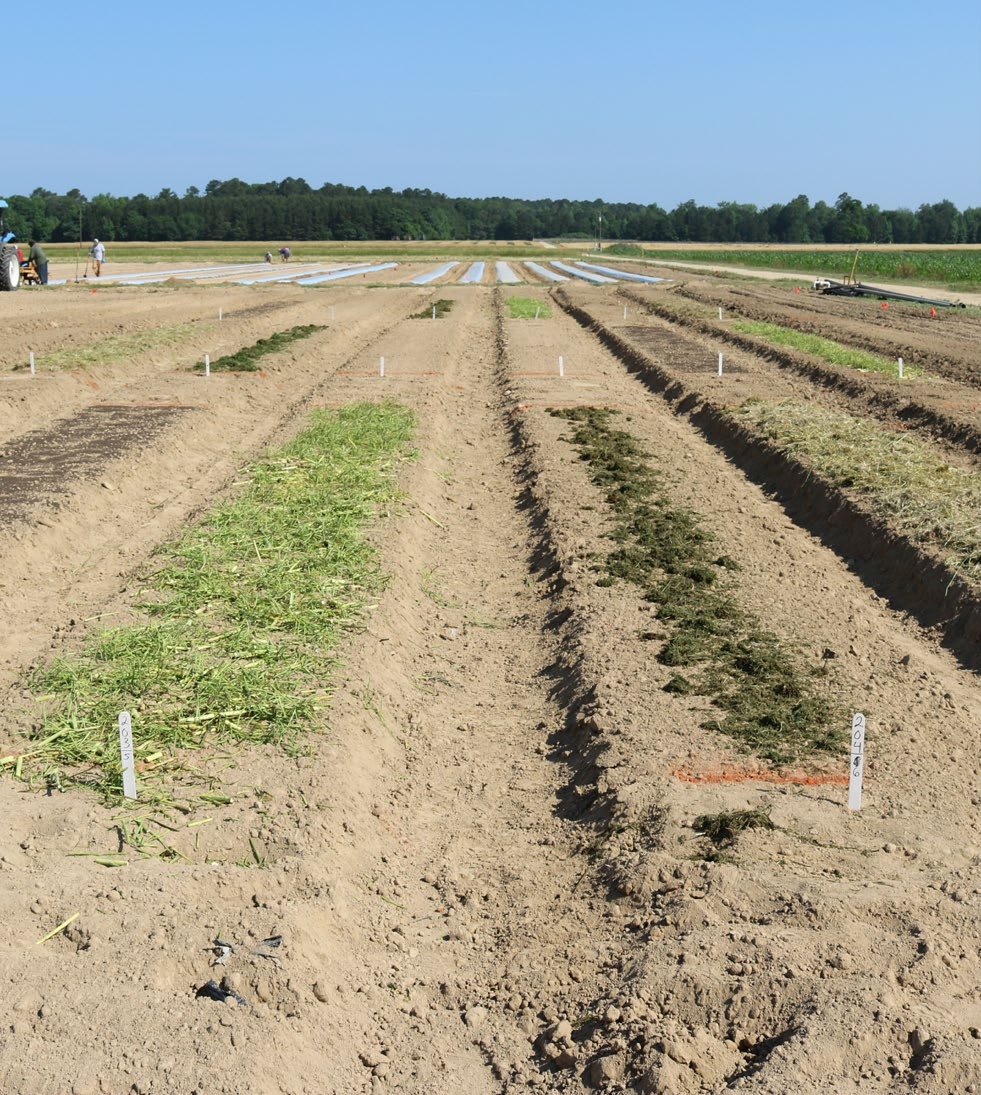
Impact on Soil Microbe Community
All soil disinfestation treatments (fumigant, physical, or ASD) have an impact on the soil microbiome. The soil microbiome, which includes bacteria, actinomycetes, oomycetes, fungi, protozoa, and nematodes, plays a key role in such important agroecosystem functions as soil organic matter decomposition and regulation of mineral nutrient availability. Like soil fumigants, ASD has broad-spectrum biocidal activity; however, the impact of ASD on soil microbial communities varies. For example, a decrease in the diversity of the bacterial community with wheat bran or molasses amendments has been reported (Testen and Miller 2018). Acidobacteria and Burkholderia populations have increased when using rice bran (Strauss and Kluepfel 2015), but Acidobacteria populations have declined when using wheat bran (Testen and Miller 2018). Thus, the type of bran also exerts an influence on the effects of the ASD treatment. Post-treatment assessments have also demonstrated changes in the diversity of the fungal community in response to ASD (Shennan et al. 2018). Ongoing research continues to assess impacts of ASD on the soil microbiome.
How to Implement ASD
Step 1. Application of carbon source: Once the carbon source and rate have been selected (see the description of carbon sources above), it is necessary to calculate the amount of carbon source for the specific area to be treated (Fig. 1). Carbon sources can be applied by hand or using equipment, such as fertilizer spreaders, when large areas are treated. Subsequently, the carbon source is incorporated into the topsoil to a depth at least of 6 to 8 inches, using a hand-pushed or a tractor-drawn rototiller.
Step 2. Covering with a plastic tarp: After incorporation of easily decomposable carbon sources, the soil is covered with plastic mulch (standard polyethylene mulch has been shown to work for ASD). Drip irrigation tape is also installed. Care must be taken to seal plastic mulch at the edges to avoid an exchange of gases (Fig. 2). Close inspection to detect plastic mulch sealing failures, followed by repairs, must be conducted.
Step 3. Irrigation application: To create anaerobic conditions, a single irrigation event to field capacity is applied. This will limit oxygen in soil by filling air pockets with water and shift soil microbial communities to facultative and obligate anaerobic respiration. The duration of irrigation will depend on the soil type, but it should be sufficient to saturate at least the top 8 inches of soil.
Step 4. ASD termination and post-treatment: ASD is terminated by allowing air to move into the soil and anaerobic volatile compounds to escape to the air, generally 2 to 4 weeks after ASD initiation. Treatment can extend longer if temperatures are cooler. Because ASD results in production of organic, volatile compounds that can be toxic to plants, planting should be delayed until at least one week after ASD termination to avoid any toxic effects on the plants. Test plants may be utilized to ensure excessive phytotoxicity will not occur.
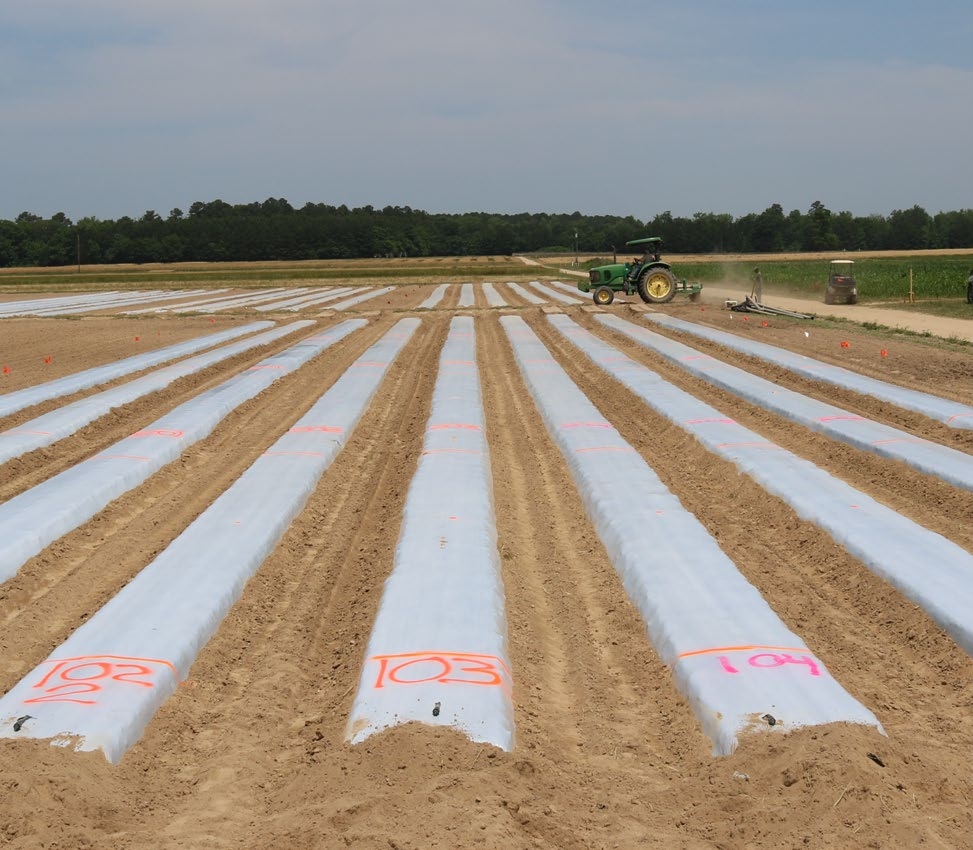
Conclusions
Studies have shown that ASD suppresses some types of soilborne pathogens, including bacteria, fungi, oomycetes, and nematodes, as well as some weeds. The effectiveness of ASD varies and depends largely on environmental conditions and carbon sources used. In Virginia, ongoing studies are evaluating ASD applications as an alternative to managing soilborne plant pathogens, as well as ASD impact on foodborne pathogens. ASD will provide vegetable producers with an ecologically sustainable alternative to soil fumigation for controlling soilborne diseases. For more information, including updated research findings consult your local Virginia Cooperative Extension office. In addition, consult the Mid-Atlantic Commercial Vegetable Recommendations VCE 456-420 for currently recommended pest control practices (https://www.pubs.ext.vt.edu/456/456-420/456-420.html).
References
Butler, D. M., Kokalis-Burelle, N., Albano, J. P., McCollum, T. G., Muramoto, J., Shennan, C., and Rosskopf, E. N. 2014. Anaerobic soil disinfestation (ASD) combined with soil solarization as a methyl bromide alternative: vegetable crop performance and soil nutrient dynamics. Plant and Soil 378:365-381.
Ibekwe, A. M., Papiernik, S. K., Gan, J., Yates, S. R., Yang, C.-H., and Crowley, D. E. 2001. Impact of fumigants on soil microbial communities. Appl. Environ. Microbiol. 67:3245-3257.
Shennan, C., Muramoto, J., Koike, S., Baird, G., Fennimore, S., Samtani, J., Bolda, M., Dara, S., Daugovish, O., and Lazarovits, G. 2018. Anaerobic soil disinfestation is an alternative to soil fumigation for control of some soilborne pathogens in strawberry production. Plant Pathology 67:51-66.
Shrestha, U., Augé, R. M., and Butler, D. M. 2016. A meta-analysis of the impact of anaerobic soil disinfestation on pest suppression and yield of horticultural crops. Frontiers in Plant Science 7:1254.
Strauss, S., and Kluepfel, D. 2015. Anaerobic soil disinfestation: A chemical-independent approach to pre-plant control of plant pathogens. Journal of Integrative Agriculture 14:2309-2318.
Testen, A. L., and Miller, S. A. 2018. Carbon source and soil origin shape soil microbiomes and tomato soilborne pathogen populations during anaerobic soil disinfestation. Phytobiomes 2:138-150.
Virginia Cooperative Extension materials are available for public use, reprint, or citation without further permission, provided the use includes credit to the author and to Virginia Cooperative Extension, Virginia Tech, and Virginia State University.
Virginia Cooperative Extension is a partnership of Virginia Tech, Virginia State University, the U.S. Department of Agriculture, and local governments. Its programs and employment are open to all, regardless of age, color, disability, sex (including pregnancy), gender, gender identity, gender expression, genetic information, ethnicity or national origin, political affiliation, race, religion, sexual orientation, or military status, or any other basis protected by law.
Publication Date
July 6, 2021