Mitigation of Greenhouse Gas Emissions in Agriculture
ID
BSE-105 (BSE-251P)
Introduction
In this publication, information is presented on how to increase farm productivity while potentially reducing greenhouse gas* (GHG) contributions from agricultural production. Some of the practices may be familiar to many producers, such as building soil organic matter (SOM) or increasing nitrogen fertilization efficiency, but many producers may not know that these same productivity-boosting activities also help to reduce GHG emissions and their impact on climate change. While informative to the producer, this publication will also inform those with an interest in both agriculture and the environmental impact of GHG emissions on the atmosphere.
*Terms defined in the glossary are italicized on first mention.
Greenhouse Gases and Climate Change
Certain kinds of human activity create GHGs, which capture heat and energy in the atmosphere and alter long-term climate cycles. The primary GHGs emitted by agriculture are carbon dioxide (CO2), methane (CH4), and nitrous oxide (N2O). Like a greenhouse that traps heat to grow tomatoes or flowers, these gases trap heat in the atmosphere and steadily increase the temperature of the Earth. A recent media release from NASA (2013) clearly shows why scientists conclude the Earth is experiencing a definite warming trend: “NASA scientists say 2012 was the ninth warmest of any year since 1880, continuing a long-term trend of rising global temperatures. With the exception of 1998, the nine warmest years in the 132-year record have all occurred since 2000, with 2010 and 2005 ranking as the hottest years on record.”
The chances are very low that the nine hottest years of record in the last 132 years should occur since 2000 simply by coincidence. Scientists agree that this evidence leads to the more plausible explanation that the Earth is actually warming and that this warming trend will continue in the foreseeable future. This is why so many scientists and engaged citizens are concerned with our GHG emissions, because by reducing them, we can also reduce the risks to our economy — including risks to agriculture — associated with climate change.
Scientists predict (to a 95 percent degree of certainty) that increased temperatures will significantly alter climate and weather patterns (Stocker et al. 2013). According to the U.S. Environmental Protection Agency (2012a), certain regions of the U.S. will become more prone to extreme weather, such as tornados, droughts, and floods. Specifically, the Eastern U.S. is expected to experience both more intense downpours during the summer, resulting in increased floods, and longer periods between these storms, resulting in increased drought. Other potential consequences of a changing climate include decreasing agricultural yields because of the rise in temperature and changes in precipitation, and the displacement of traditional crops northward, which may force producers to change the crops they can grow in order to adapt to the new climate.
The Global Warming Potentials of Different GHGs
Greenhouse gases have very different potency in the atmosphere. The potency of a GHG is referred to as its global warming potential and is commonly expressed as a carbon dioxide equivalent (CO2e). The potency of three common GHGs is shown in table 1. Methane and nitrous oxide are much more potent than carbon dioxide; that is, their presence in the atmosphere is considerably more harmful than CO2 alone.
Greenhouse gas | Global warming potential |
---|---|
Carbon dioxide (CO2) | 1 CO2e |
Methane (CH4) | 21 CO2e |
Nitrous oxide (N2O) | 310 CO2e |
(Source: “Overview of Greenhouse Gases,” U.S. EPA;
https://www.epa.gov/ghgemissions/overview-greenhouse-gases.)
How Does Agriculture Contribute to Climate Change?
According to the U.S. Environmental Protection Agency (U.S. EPA 2012b), on-farm GHG emissions contribute 8 percent of all U.S. emissions (530 million metric tons of CO2 equivalent; fig. 1). On-farm GHG sources include N2O emissions from nitrogen fertilizer and cropping practices; CH4 generation from livestock digestion, also known as enteric fermentation; and manure; as well as CO2 from fossil fuels used to operate farm machinery, irrigation pumps, and heaters. However, the agriculture industry as a whole creates more GHGs than those created by farms. Fossil fuels are used to produce inorganic fertilizers via the Haber-Bosch process, to manufacture herbicides and pesticides, for seed production, and in postharvest transport and storage.
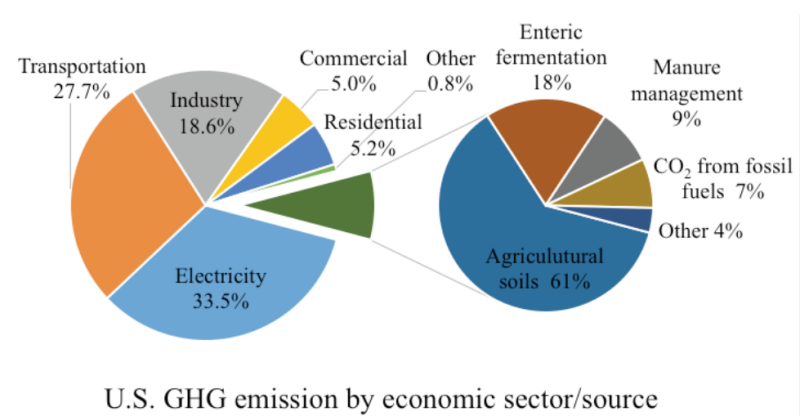
Additionally, agriculture contributes indirectly to GHG emissions through land use change. Healthy forests remove GHGs from the atmosphere. Therefore, clearing forests for agriculture will increase net GHG emissions. In fact, some recent estimates state that the entire agricultural industry contributes up to a third (12,000 megatons or 12 trillion tons) of all GHGs globally (Gilbert 2012).
Ways to Mitigate GHGs in Agriculture
While agriculture may contribute a significant amount of GHGs, there is great potential for GHG mitigation in agriculture. There are a number of approaches that could result in beneficial, efficient, and cost-effective farm management practices that simultaneously lower net GHG emissions. While there is a wide range of management and environmental factors that can affect both productivity and GHG emissions on farms, the following suggestions can help protect a producer’s bottom line along with protecting the environment. Figure 2 provides an illustration of the sources and sinks of GHGs in agriculture. The following sections provide suggestions on how producers can mitigate these sources and enhance sinks. Table 2 provides a synopsis of these suggested practices.
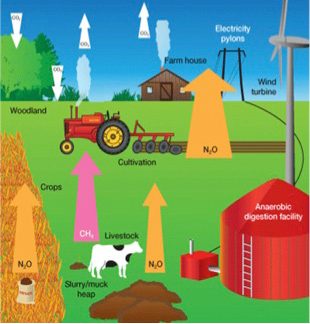
Activity | Benefit to farmers | Benefit to the environment |
---|---|---|
Increasing SOM | Benefits soil fertility and crop productivity. | Provides a sink for CO2. |
Increasing fertilization efficiency | More fertilizer being used by crops, so less is wasted. | Reduces N2O — the largest contributor of greenhouse gases from farms. |
Manure management | Applying manure at the right time and place allows more nutrients to go to the crops, so less is wasted. Anaerobic digestion provides a source of income and energy. | Applying manure at the right time reduces N2O and water pollution. Anaerobic digestion provides renewable energy source for farms, reduces CH4. |
Planting trees | Source of energy, reduces heat stress on livestock, and provides erosion and flood control. | Provides a sink for CO2. |
Renewable energy production | Source of income and energy. | Reduces net CO2 emissions from farms. |
Crop Production
Soil Organic Matter and Carbon Dioxide
According to the U.S. Department of Agriculture Natural Resources Conservation Service (USDA-NRCS 2014), soil organic matter increases soil moisture and nutrient retention while improving soil structure. These soil attributes are well-known positive properties and are strongly correlated to high crop productivity. Increasing SOM will also serve as a CO2 sink, meaning that increasing SOM in a field will actually sequester (remove) CO2 from the atmosphere, mitigating a farm’s GHG footprint. Thus, boosting a farm’s crop productivity through increased SOM can also help to lessen the impacts of climate change.
There are two common ways to increase SOM. The first is to employ reduced tillage or no-tillage agriculture, and the second is to use continuous cropping with increased residues. No-tillage or reduced tillage has been clearly shown to increase SOM, while conventional plow-tillage has been shown to decrease it. Tilling a field exposes SOM to oxidation and makes it more suscepti- ble to erosion — both of which cause SOM depletion. Advances in seed technology, pest control, and farm machinery are making no-tillage and reduced tillage more acceptable to producers (see “Successful No-Tillage Corn Production,” VCE publication 424-030; and “No-Tillage Small Grain Production in Virginia,” VCE publication 424-005). Cropping sequences that include a fallow period tend to reduce SOM, as compared to continuous cropping, which tends to increase SOM. Cover crops and nitrogen-fixing legumes are often recommended to both enhance fertility and increase SOM (see “Building Soil Organic Matter With Cover Crops,” VCE publication 2906-1381; and “Building Healthy Soils,” VCE publication 426-711).
Fertilization and Nitrous Oxide
While the mitigation of CO2 in agriculture lies in the conservation and enhancement of SOM, opportunities to mitigate N2O exist by incorporating precision agriculture techniques into production practices. Several studies have shown that nitrogen fertilizer applied in excess of crop needs does not increase yields and can significantly increase N2O production; excess fer- tilizer not utilized by crops is made available to soil microorganisms, which increases N2O production. This excess fertilizer, in conjunction with irrigation or rain events, results in even greater N2O production and potential leaching. In summary, producers can reduce both nutrient leaching and GHG emissions by optimizing nitrogen fertilizer placement, timing, and application rate. Local producers should work with Virginia Cooperative Extension agents and specialists in order to increase fertilization efficiency, remain profitable, and ultimately reduce their N2O footprint (see “Nitrogen Soil Testing for Corn in Virginia,” VCE publication 418-016; “Nitrogen and Phosphorus Fertilization of Corn,” VCE publication 424-027; and “Denitrification Management,” VCE publication BSE-54P).
Animal Production
Manure Storage: Nitrous Oxide and Methane
Opportunities exist to control CH4 and N2O production from animal agriculture by optimizing the use of manure and improving animal diets. Proper manure management is one way for a producer to reduce GHG emissions and improve production efficiency. Proper storage of manure allows the producer to apply this resource during optimal weather and field conditions, which reduces fertilization expenses and increases fertilization efficiency. As an added benefit to the environment, water and air pollutants (e.g., nutrients and N2O) are mitigated (see “Selection and Location of Poultry and Livestock Manure Storage,” VCE publication 442- 307; and “Manure Management and Environmental Stewardship,” VCE publication 442-309). While prolonged manure storage will naturally result in greater cumulative GHG production, frequent turning of the manure pile will reduce emissions because constant incorporation of oxygen will disrupt N2O and CH4 production within the pile.
Animal Diet: Nitrous Oxide and Methane
Improving animal diets by maintaining pastures and optimizing nutrition can also reduce GHG production. Maintaining pasture quality will increase livestock production and decrease energy loss from CH4 emissions. Pasture quality can be improved by reducing the overall fiber content, making it easier for livestock to digest. For instance, the incorporation of legumes and alfalfa in pasture has been shown to decrease CH4 emissions in livestock. Replacing foraging with concentrates and grains will also decrease CH4 emissions. Finally, protein optimization can reduce N2O emissions by controlling how much nitrogen animals ingest.
Anaerobic Digestion: Methane
Anaerobic digestion of manure for energy production both reduces GHG emissions and provides important benefits to farms. These benefits include on-farm energy production, increased revenue, and fertilizer for soil amendments. Revenue is provided by excess electricity, manure sales, and reuse of digested solids. Because methane is used for energy production and is not emitted into the atmosphere, this practice will reduce a farm’s GHG emissions (see “Biomethane Technology,” VCE publication 442-881).
Energy Conservation and Renewable Energy Sources
Trees and Woodlots
Integrating woodlots on farms can provide several benefits to producers while reducing GHG emissions. First, trees can remove substantial amounts of CO2 from the atmosphere, incorporating it into woody biomass and root systems. Trees can also be a renewable energy source. They can be used for heat, and more trees can be planted in their place in order to continue providing economical and sustainable energy for the farm. Ideally, woodlots would contain trees at different stages of growth. This would ensure that trees continue to utilize CO2 while also providing energy for the farm. Trees can also provide shade and shelter, reducing heat stress on livestock and increasing productivity by creating healthier conditions. Trees also reduce wind stress on buildings, which can cut energy requirements for heating.
Alternative Fuels
Plant-based biofuels (e.g., ethanol), when used as fuel, release CO2 into the environment, but do not increase net atmospheric CO2 levels. This is because the CO2 released during use is sequestered in the same year by the growth of new biomass used to make the ethanol or biofuel. U.S. farms have the potential to produce significant quantities of ethanol or plant-based biofuels that could be sold on the energy market or utilized in their own production operations.
On-Farm Energy Production (wind, solar, geothermal)
Because farms occupy a significant area of the U.S. land base, opportunities exist for producers to supplement their income, reduce energy consumption from the grid, and reduce GHG emissions by investing in alternative energy sources such as wind, solar, and geothermal. While all three of these alternative energy sources represent significant upfront investment costs for the infrastructure, the ultimate source of the energy is free (e.g., sunlight, wind, and heat stored in the earth) and thus, over the long term, can provide considerable reductions in energy costs to producers (USDA 2010).
Often, government agencies such as the USDA or the Department of Energy provide subsidies to install alternative energy sources. The USDA (2010) brochure “Advanced Renewable Energy” supplies information about the subsidies available to producers and is available at https://www.usda.gov/energy/maps/resources/brochure/$file/renewable_energy_brochure.pdf.
The Bottom Line
By investing in sound farming practices, a producer can actually increase crop productivity and profitability while also reducing its long-term economic risk from the harmful effects of climate change. In other words, by helping themselves, producers will be helping the environment and their bottom line.
Glossary
Anaerobic digestion – A process that breaks down organic material (e.g., manure) by microorganisms in the absence of oxygen.
Carbon dioxide (CO2) – A gaseous product of fossil fuel combustion that contributes to climate change when released into the atmosphere.
Climate change – The aggregated effects of increased temperatures and their role in changing climate and weather patterns.
Enteric fermentation – A digestive process by which carbohydrates are broken down by microorganisms for absorption by the animal, sometimes releasing methane.
Greenhouse gases – A collection of human and naturally derived gases that trap heat within the atmosphere and contribute to climate change.
Haber-Bosch process – The process of converting atmospheric nitrogen gas (N2) into inorganic nitrogen fertilizers.
Methane (CH4) – A gaseous product of animal husbandry that contributes to climate change when released into the atmosphere.
Mitigation – Making an activity less harmful by minimizing the source of harm.
Nitrous oxide (N2O) – A gaseous product of denitrification and nitrification from agricultural fields that contributes to climate change when released into the atmosphere.
Oxidation – The conversion of matter (e.g., soil organic matter) to a less beneficial form by exposure to oxygen.
Precision agriculture – Optimizing productivity and efficiency with the use of scientific information and methods, often employing advanced technology. For example: Using weather prediction applications on hand-held devices to plan when it’s best to apply fertilizer.
Sequester – To remove from or make unavailable.
Sink – Climate change language for a process that removes greenhouse gases (e.g., building up soil organic matter and planting trees).
Soil organic matter – Plant and animal residues, cells, and tissues or soil organisms and the substances the organisms synthesize.
Related Virginia Cooperative Extension Publications
Alley, M. M., M. E. Martz Jr., P. H. Davis, and J. L. Hammons. 2019. Nitrogen and Phosphorus Fertilization of Corn. Virginia Cooperative Extension Publication 424-027.
https://www.pubs.ext.vt.edu/content/dam/pubs_ext_vt_edu/424/424-027/424-027.pdf.
Easton, Z. M., and E. M. Lassiter. 2018. Denitrification Management. Virginia Cooperative Extension Publication BSE-54P.
http://digitalpubs.ext.vt.edu/vcedigitalpubs/3145838677287997/MobilePagedReplica.action?pm=2&folio=1#pg1.
Evanylo, G. K., and M. M. Alley. 2019. Nitrogen Soil Testing for Corn in Virginia. Virginia Cooperative Extension Publication 418-016.
https://www.pubs.ext.vt.edu/418/418-016/418-016.html.
Lassiter, E. M., and Z. M. Easton. 2018. Denitrifying Bioreactors: An Emerging Best Management Practice to Improve Water Quality. Virginia Cooperative Extension Publication BSE-55P.
https://www.pubs.ext.vt.edu/content/dam/pubs_ext_vt_edu/BSE/BSE-55/BSE-227.pdf.
Ogejo, J. A. 2018. Poultry and Livestock Manure Storage: Management and Safety. Virginia Cooperative Extension Publication 442-308.
https://www.pubs.ext.vt.edu/content/dam/pubs_ext_vt_edu/442/442-308/BSE-244.pdf.
Ogejo, J. A. 2018. Selection and Location of Poultry and Livestock Manure Storage. Virginia Cooperative Extension Publication 442-307.
https://www.pubs.ext.vt.edu/content/dam/pubs_ext_vt_edu/442/442-307/BSE-243.pdf.
Ogejo, J. A. 2018. Manure Management and Environmental Stewardship. Virginia Cooperative Extension Publication 442-309.
https://www.pubs.ext.vt.edu/content/dam/pubs_ext_vt_edu/442/442-309/BSE-245.pdf.
Ogejo, J. A., Z. Wen, J. Ignosh, E. Bendfeldt, and E. R. Collins Jr. 2018. Biomethane Technology. Virginia Cooperative Extension Publication 442-881.
https://www.pubs.ext.vt.edu/content/dam/pubs_ext_vt_edu/442/442-881/BSE-240.pdf.
Prunty, R. 2009. Building Soil Organic Matter With Cover Crops. Virginia Cooperative Extension Publication 2906-1381. http://pubs.ext.vt.edu/2906/2906-1381/2906-1381_pdf.pdf.
Relf, D. 2017. Building Healthy Soil. Virginia Cooperative Extension Publication 426-711.
https://www.pubs.ext.vt.edu/content/dam/pubs_ext_vt_edu/426/426-711/426-711_HORT-244Pv2.pdf.
Thomason, W. E., M. M. Alley, E. L. Stromberg, E. S. Hagood, and A. Herbert. 2009. No-Tillage Small Grain Production in Virginia. Virginia Cooperative Extension Publication 424-005.
http://pubs.ext.vt.edu/424/424-005/424-005_pdf.pdf.
Thomason, W. E., R. R. Youngman, E. S. Hagood, E. L. Stromberg, and M. M. Alley. 2019. Successful No-Tillage Corn Production. Virginia Cooperative Extension Publication 424-030.
https://www.pubs.ext.vt.edu/cotent/dam/pubs_ext_vt_edu/424/424-030/424-030.pdf.
Additional Resources
Farming Futures. 2009. Climate Change: Be Part of the Solution – Focus On: Nutrient Management. Farming Futures Fact Sheet No. 21.
http://adlib.everysite.co.uk/resources/000/241/422/FS21.pdf.
Farming Futures. 2009. Climate Change: Be Part of the Solution – Focus On: Soil Management. Farming Futures Fact Sheet No. 20.
http://www.nutrientmanagement.org/assets/12060.
Farming Futures. 2009. Climate Change Series: General Opportunities and Challenges. Farming Futures Fact Sheet No. 2.
References
Farming Futures. 2017. Greenhouse Gases Explained. www.farmingfutures.org.uk/resources/greenhouse-gases-explained.
Gilbert, N. 2012. “One-Third of Our Greenhouse Gas Emissions Come From Agriculture.” Nature, October 31. www.nature.com/news/one-third-of-our-greenhouse-gas-emissions-come-from-agriculture-1.11708.
NASA. 2013. “NASA Finds 2014 Sustained Long-Term Climate Warming Trend.” Media Release No. 13-021. https://www.nasa.gov/content/god-dard/nasa-finds-2013-sustained-long-term-climate-warming-trend/.
Stocker, T. F., D. Qin, G.-K. Plattner, M. Tignor, S. K. Allen, J. Boschung, A. Nauels, Y. Xia, V. Bex, and P. M. Midgley (eds.). 2013. “Summary for Policymakers.” In Climate Change 2013: The Physical Science Basis. Contribution of Working Group I to the Fifth Assessment Report of the Intergovernmental Panel on Climate Change (IPCC). Cambridge, United Kingdom: Cambridge University Press.
https://www.researchgate.net/profile/Abha_Chhabra2/publication/271702872_Carbon_
and_Other_Biogeochemical_Cycles/links/54cf9ce80cf24601c094a45e/Carbon-and- Other-Biogeochemical-Cycles.pdf.
USDA (U.S. Department of Agriculture). 2011. Advanced Renewable Energy. USDA Brochure.
https://www.usda.gov/energy/maps/resources/brochure/$file/renewable_energy_brochure.pdf.
USDA-NRCS (U.S. Department of Agriculture Natural Resource Conservation Service). 2014. “Role of Organic Matter in Soil.” USDA Web Page.
www.nrcs.usda.gov/wps/portal/nrcs/detailfull/soils/health/mgnt/?cid=nrcs142p2_053859.
U.S. EPA (U.S. Environmental Protection Agency). 2012a “Climate Impacts in the Southeast.” EPA Web Page. https://19january2017snapshot.epa.gov/climate-impacts/climate-impacts-southeast_.html.
U.S. EPA (U.S. Environmental Protection Agency). 2012b “Sources of Greenhouse Gas Emissions.” EPA Web Page. https://www.epa.gov/ghgemissions/sources-greenhouse-gas-emissions.
Virginia Cooperative Extension materials are available for public use, reprint, or citation without further permission, provided the use includes credit to the author and to Virginia Cooperative Extension, Virginia Tech, and Virginia State University.
Virginia Cooperative Extension is a partnership of Virginia Tech, Virginia State University, the U.S. Department of Agriculture, and local governments. Its programs and employment are open to all, regardless of age, color, disability, sex (including pregnancy), gender, gender identity, gender expression, genetic information, ethnicity or national origin, political affiliation, race, religion, sexual orientation, or military status, or any other basis protected by law.
Publication Date
April 3, 2019