Virginia Master Naturalist Basic Training Course: Botany
ID
ANR-10NP (CNRE-166NP)
Objectives
- Describe the diversity and distribution of plants in Virginia.
- Describe the role of plants in Virginia ecosystems.
- Describe the natural history and basic biology of plants.
- Describe the adaptations of plants and how these relate to environmental factors.
- Describe the taxonomy of plants.
- Describe the key characteristics used to identify plants.
- Describe threats and/or issues relating to plants in Virginia, such as invasive species.
- Recognize some common plants occurring in the local ecosystems.
- Recognize rare or special species that indicate habitat qualities.
- Understand appropriate techniques and methods for studying plants.
Diversity and Distribution of Plants in Virginia
Diversity
Plants can be defined as multicellular, photosynthetic organisms with reproductive structures that are more complex than single cells. By this definition, algae are not considered plants because they are either unicellular or their reproductive structures are essentially unicellular. Fungi, too, are excluded because they are not photosynthetic. At least 400 million years of diversification has resulted in a wide diversity of taxonomically distinct major groups of plants. Some of the most important groups of plants found in Virginia are described below.
Vascular Plants
Vascular plants are internally complex with specialized conductive tissues, such as xylem and phloem.
- Angiosperms or flowering plants (fig. 1) – Most trees, shrubs, and herbs encountered in nature (or in cultivation) belong to this, the youngest and most recently evolved group of plants. They can be characterized as reproducing by flowers in a process that involves pollen, ovules, and seeds; the ovules develop into seeds while contained within an ovary, which matures into a fruit. There are approximately 3,500 species of flowering plants that occur spontaneously in the Commonwealth of Virginia, approximately 25 to 30 percent of which are native to other regions of the world but now live here, too.
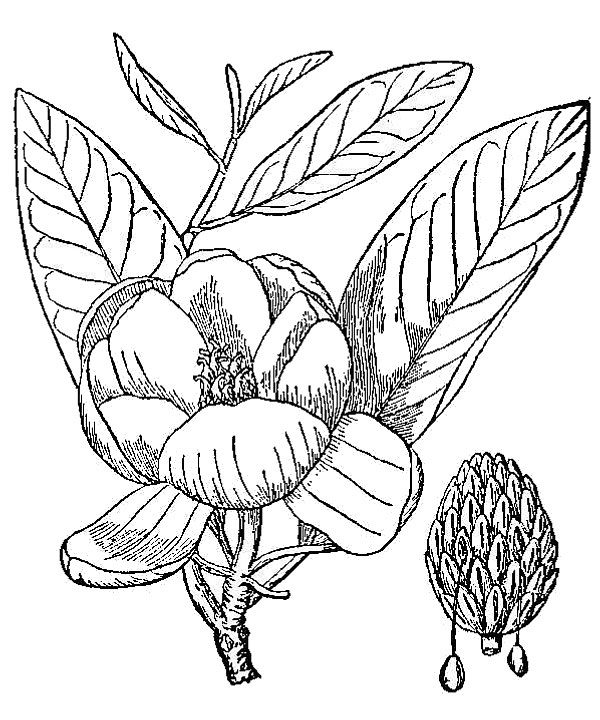
- Conifers (fig. 2) – These are the cone-bearing, woody plants with scale- or needle-like leaves. They also reproduce via pollen, ovules, and seeds, but the ovules and seeds develop as part of a cone organ that leaves the ovules directly exposed to the environment — at least for part of their development. Thus, conifers are one group of gymnosperms (literally, plants with “naked seeds”). Other living gymnosperm groups include the cycads, Gnetophytes, and Ginkgo; of these, only Ginkgo — or maidenhair tree, native to China — is commonly encountered in cultivation in Virginia. There are 22 species of conifer native to Virginia; about a dozen other species can be found in cultivation.

- Ferns (fig. 3) – Ferns are vascular plants that do not reproduce via pollen, ovules, and seeds. Instead, familiar fern plants make multicellular sporangia that release dust-like unicellular spores. Fern spores grow into a small and easily overlooked gametophyte generation within which eggs are fertilized by swimming sperm cells. The resulting zygote eventually grows into the familiar fern plant, which represents the sporophyte generation. Horsetails (genus Equisetum; fig. 4) are now classified among the ferns. There are nearly 80 fern species native to Virginia, as well as some 14 naturally occurring hybrids and perhaps four naturalized species.
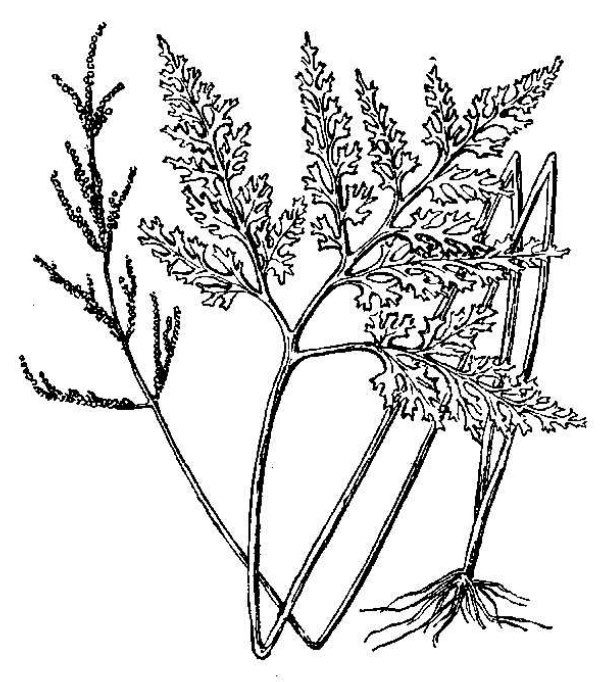

- Club mosses (fig. 5) – In general terms, club mosses are reasonably similar to ferns, but they differ in many details: the leaves are smaller, often scale-like, and have just a single unbranched vein; the sporangia are grouped in a cone-like strobilus; and the internal tissue layers are organized differently. Until recently, club mosses were classified in a single genus, Lycopodium. Virginia has 14 club moss species and four naturally occurring hybrids, now classified by some taxonomists in seven genera.
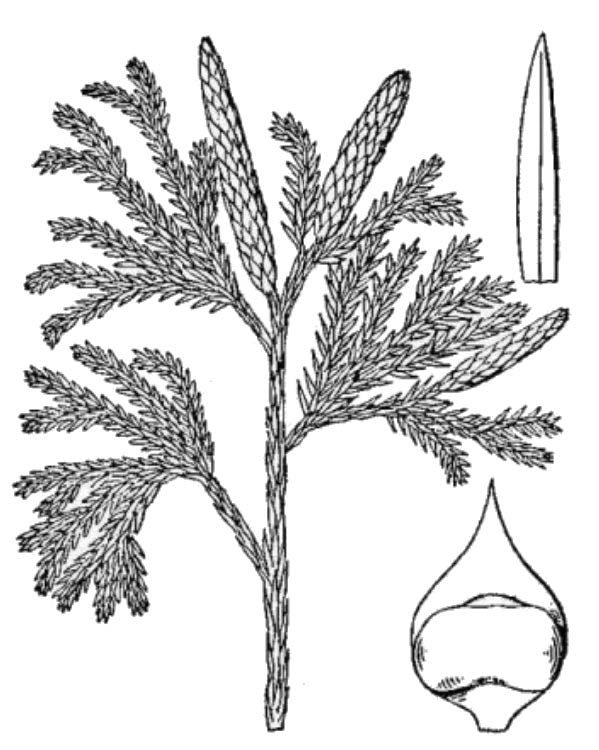
- Spikemosses (fig. 6) – Spikemosses are like delicate club mosses, but they differ by making two different kinds of spores (heterospory) and by the presence of a microscopic ligule associated with each minute leaf. There are two species of Selaginella (spikemoss) native to Virginia.

- Quillworts (fig. 7) – At first glance, quillworts look like a tuft of grass growing in or near water. Although they don’t look much like spikemosses, these groups are related as attested by the shared presence of ligules and two types of spores. There are 11 species and four hybrids of Isoetes (quillwort) native to Virginia.

Nonvascular Plants
Nonvascular plants have relatively simple internal organization with neither xylem nor phloem present.
- Mosses (fig. 8) – Mosses tend to grow in moist places or have the ability to go dormant when dry. The familiar small, leafy moss plant is the gametophyte generation, and it periodically produces a spore-bearing capsule atop a thin bristle-like stalk.

- Hornworts (fig. 9) – These obscure, small plants consist of a sheet-like patch of cells on moist ground that eventually forms cylindrical, spore-bearing spikes.

- Liverworts (fig. 10) – Liverworts generally consist of flat, ribbon-like plants that grow horizontally on moist surfaces, but there is one group that superficially resembles a creeping, leafy moss plant (these never have a leaf midrib, which mosses always have). Like mosses, liverwort plants consist of the gametophyte generation; the spore-bearing structures can resemble those of mosses but differ in detail.

Distribution
One of the first things we notice is that plant species are not distributed uniformly in the environment. Some are common and widespread, while others are much less frequent or even rare. Plants don’t necessarily grow wherever a seed happens to land. Instead, the same species tend to occupy places with similar environmental conditions: swamp forest, old field, limestone rock outcrop, etc. In other words, every species of plant has its own particular environmental requirements for survival.
A plant can exist in a particular spot only if temperature, moisture, nutrient availability, and interactions with other organisms (to name just a few of these factors) permit its survival. Seldom do the factors controlling plant distribution act in isolation, e.g., elevation is correlated with length of growing season, average daily temperature, and daily temperature extremes. On Virginia mountaintops, growing seasons are shorter, temperatures are cooler, and day-to-night temperature fluctuations are greater than what a plant would encounter on the shores of the Chesapeake Bay.
Geological factors — including the type of bedrock and how it weathers to soil — can influence plant distribution, especially in terms of soil depth, nutrient content, pH, water-holding capacity, and presence (or absence) of heavy metals. As a general rule, limits of tolerance are defined by extreme conditions rather than average values. Therefore, the coldest winter temperature has a greater influence on plant distribution than the average winter temperature.
Extreme low winter temperatures vary with latitude and altitude and constitute the basis for the U.S. Department of Agriculture’s plant hardiness zones — in essence, a system of geographic zones that share similar extreme winter cold temperatures, mapped in 10-degree intervals. Virginia occupies portions of USDA zones 5 (high mountains), 6 (most of the state), and 7 (the Tidewater region).
Some plants have very narrow environmental tolerances and other plants are much wider in their adaptability. Some species are considered to be generalists because they tolerate a wide variety of conditions, allowing them to occupy a diversity of habitats. In ecological parlance, we would say they have broad ecological amplitude. Thus, in terms of plant distribution within Virginia, some species occur essentially throughout the state and well beyond. For example, red maple (Acer rubrum) and Jack in the pulpit (Arisaema triphyllum) can be found throughout Virginia, whereas other plants are much more limited in their distribution.
Restricted distributions — as opposed to statewide occurrence — often correlate well with the major physiographic regions of the state. Thus, plants like summer sweet (Clethra alnifolia) occur mainly in the coastal plain; Hexastylis lewisii is found only in the Piedmont (and just the southern portion, at that); and the flame azalea (Rhododendron calendulaceum) and rose bay (R. catawbiense) are primarily restricted to the mountains.
Other species might only rarely be found outside of a narrow set of conditions and thereby are limited geographically by the frequency of those conditions in the landscape. Virginia sneezeweed (Helenium virginicum) — restricted to a few sinkhole ponds in the Shenandoah Valley and similar habitats in Missouri — is a good example of a rare species with narrow ecological amplitude.
Some plant species widespread to the North reach their southern limit in the mountains of Virginia. Some of these plants [for example, Sibbaldiopsis (Potentilla) tridentata] probably reached Virginia from the North while retreating from the advancing Pleistocene glaciers and now persist in the mountains as relicts. Other species widespread in the Southeastern U.S. reach their northern limit in the Tidewater region, where zone 7 climatic conditions prevail.
A major resource for study of plant distribution in the state is the ‘Digital Atlas of the Virginia Flora” (www.vaplantatlas. org/). This website maps the known occurrence, county by county, of all species of vascular plants found in Virginia. Each county occurrence is documented by at least one specimen preserved in a museum (herbarium) collection. For each species included, the atlas also indicates whether it is a native member of the Virginia flora or introduced from elsewhere.
Climate change has always been an important factor in plant distribution. When climate change brings about unfavorable conditions, plants have two options: (1) adapt/evolve to accommodate the new conditions or (2) migrate (for example, by seed dispersal) to colonize new regions that match their requirements. While it seems clear that some degree of human-induced climate change is occurring or will soon occur, there is no certainty about the rate at which that change will take place or how extreme its full extent will be. Climate change presents rare plants with especially large challenges. It remains to be seen whether rare plants have sufficient genetic diversity to adapt in place or if they can produce enough seeds to colonize efficiently favorable sites for growth in a changing landscape.
Most commonly, plant species are associated with specific soil types or substrates, certain hydrological conditions, sun or shade, and disturbed or undisturbed conditions. The degree to which a species adheres to these parameters varies, of course, across the full spectrum. As a consequence, the patterns that emerge are complex and tend to be fuzzy.
Observations of a species over its whole geographic range help to develop a concept of its “preferred” habitat. These observations will often include common associates — other species occupying the same or similar environmental conditions. These associations, along with their defining environmental parameters, may be recognized on a landscape scale as plant communities. Communities that are not greatly affected by the activities of man are called natural communities. The process of delimiting and classifying natural communities is an ongoing process, but Virginia is fortunate to have an excellent resource in “The Natural Communities of Virginia: Ecological Groups and Community Types” (Fleming and Patterson 2012), which provides descriptions as well as typical or defining plant species for each community type. Because plants are typically the most conspicuous and stable biotic component of a natural community, they are an important factor in describing and characterizing it.
In the same way that plant species vary in abundance in the landscape, natural communities vary in their frequency. To choose an example familiar to nearly everyone, consider a coastal beach or strand. The physical parameters in this case frame a rather extreme and harsh environment for most plant species. The substrate is sand — loose and shifting by the action of wind and waves, creating a naturally disturbed and unstable habitat. Saltwater and salt spray require special adaptations for water uptake and to prevent desiccation.
The leaves of a plant from the coastal strand community are often succulent or tough and in-rolled. The open, windy conditions favor the wind-pollinated grasses such as sea oats (Uniola paniculata), beach grass (Ammophila breviligulata), and saltmarsh cordgrass (Spartina patens). We find searocket (Cakile edentula) and Carolina saltwort (Salsola caroliniana) on the beach. In one sense, the habitat is rare in that it is limited to the near-shore environment, but it is geographically widespread, and many of its plant components range all the way up and down the East Coast and well beyond.
Role of Plants in Virginia Ecosystems
Plants have many ecological roles. Plants constitute the base of the food chain. The vast majority of plants are photosynthetic: They use sunlight to synthesize sugar from carbon dioxide and water via a complicated series of reactions. These sugar molecules provide both the energy and chemical starting material for plant metabolism and growth. In essence, plants make their own food; therefore, in ecological terms, they are often characterized as autotrophic (literally, self-feeding).
In most familiar ecosystems, plants ultimately provide the food consumed by all other organisms: Herbivores eat plants, and carnivores eat the herbivores that once ate plants. And when plants die or shed dead leaves or flakes of bark, etc., microorganisms (e.g., bacteria and fungi) derive their sustenance from these once-living plants or plant parts.
Plants require more than water and carbon dioxide to survive, however. Plant roots absorb up to 14 different elements from the soil and incorporate them into the biochemistry of their cells: phosphorus, potassium, nitrogen, sulfur, calcium, iron, magnesium, boron, manganese, nickel, copper, zinc, molybdenum, and chloride. Other forms of life require these elements, too, and ultimately these elements enter the biosphere largely by way of plants.
Finally, it is of vital importance that plants release oxygen as a byproduct of the photosynthetic process. Ultimately, every breath we take, every breath of every animal, and every passage of water over gills serves to deliver life-giving oxygen made and continually renewed by plants. In summary, plant photosynthesis captures energy and builds nutritious tissues that eventually feed all other organisms. In doing so, plants also release oxygen, which the efficient utilization of that food energy depends on, for plants, animals, and human beings, too.
Plant life defines ecological communities. Forest, marsh, meadow, and bog are recognizably distinct in large part because of the nature of their plant life. The presence or absence of certain plants determines not only the overall appearance of any given habitat, but it also dictates much about food availability, nesting sites, and hiding places for animals. Plants create multiple niches within any given community. Consider, for example, the different environmental aspects exploited by animals that live in a forest canopy versus those that occupy the forest floor or those that burrow into the soil and live among the roots of trees.
Natural History and Basic Biology of Plants
While plants and animals share a great many fundamental aspects of their biology, they also differ in profound ways. Perhaps one of the most obvious differences is that plants are sessile (nonmotile) because their roots anchor them. In contrast, many animals are capable of locomotion. While animals appear active, dynamic, and engaged in all manner of fascinating behaviors, plants seem static and nonchanging on first impression.
That impression, of course, is an artifact of our perception of time. Plants are dynamic; they undergo growth and change, but their rates of change are usually too slow for impatient humans to take much notice. Nevertheless, it is common knowledge that an apple tree will be leafless in winter, covered in flowers in early spring, leafy all summer, and bear delicious ripe apples in the fall. These seasonal transformations are profound; further, as the years go by, that apple tree greatly increases its size, far surpassing the growth capacity of most animals. Plants are indeed dynamic!
Familiar terrestrial animals have a determinate body plan: After birth or hatching, they grow to a certain size and attain a stereotyped form that is characteristic of their particular species. All monarch butterflies are very nearly the same size, and they all have six legs and four wings, etc.
Plants, on the other hand, have an indeterminate body plan: Their overall size and growth form depend to a large degree on age, the environment, and chance factors. No two maple trees in a forest will have exactly the same height, trunk diameter, or number of branches, even if they happen to be of the same age.
To a large degree, the indeterminate body plan of plants is best understood as a consequence of their fundamental mode of growth via meristems. A meristem is a group of plant cells with a continuing long-term capacity for cell division and growth. Details of meristem activity are described elsewhere in this chapter, but the important point here is that meristems allow plants to grow by the addition of new cells, tissues, and organs to the plant body over extended periods of time — years, decades, centuries, or millennia, depending on the plant species.
Reproduction
Despite their sedentary nature, populations of plants are capable of dispersal and migration across the landscape, usually in the form of seeds, seed-bearing fruits, or spores (in the case of mosses, ferns, and similar plants), not by migration of individual mature plants! Again, it is useful to think about plant dispersal in relatively long time frames as generation after generation of a particular species disperses its seeds. Of course, large jumps covering vast distances, though rare, can happen; consider the native plants of Hawaii that reached that oceanic island via long-distance dispersal.
Seeds are fundamental to the reproduction of many plants, and reproduction is one of the general characteristics of all living things. There are certain fundamental similarities about the nature of sexual reproduction in plants and animals, but there are also some profound differences.
At its core, sexual reproduction involves cellular mechanisms that generate subtle alterations in the genetic constitution of offspring through successive generations. At the cellular level, the central features of sexual reproduction are meiosis and syngamy. Meiosis starts with genetically similar diploid cells (containing two copies of genetic information) and yields genetically diverse haploid cells (containing just single copies of genetic information). Syngamy (or fertilization) amounts to a random recombination of these haploid cells to restore the diploid condition but with somewhat different combinations of genes than found in either parent. In animals, the process is relatively familiar: Meiosis produces cells that function directly as gametes; females make eggs and males make sperm. The union of sperm and egg forms a zygote, the first cell of the next generation offspring.
Plants are more complicated. Diploid plants (sporophytes) make spores by meiosis, spores grow into haploid plants (gametophytes), and gametophytes make gametes (eggs and sperm) that unite to form a zygote — the first cell of the next sporophyte generation. In the case of relatively simple plants, the gametophyte and sporophyte generations are easily observed as distinct phases in an alternation of generations life cycle (see figs. 8 and 9). In seed plants (fig. 11), the male gametophyte generation is contained within pollen, and the female gametophyte is a mere developmental stage found within ovules that eventually mature as seeds containing an embryo — an immature new sporophyte generation.
A college-level biology or botany textbook should be consulted to further explain and explore the significance of the alternation of gametophyte and sporophyte generations in plant reproduction.
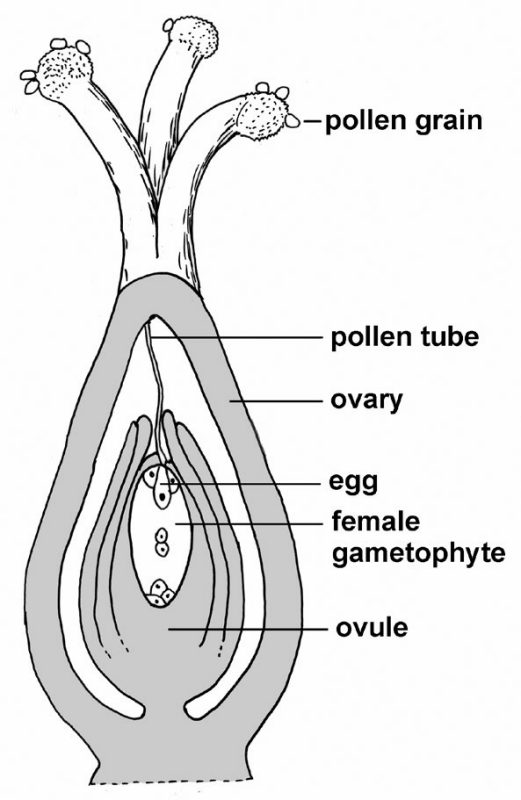
Physiology
One of the subtle differences between plants and animals has to do with nitrogen metabolism. In general, animals are relatively limited in their biochemical capacity to process molecules containing nitrogen. Consequently, animals generate significant amounts of nitrogen-based waste products that must be excreted either as ammonia, urea, or uric acid.
Plants, in contrast, are much more versatile in their biochemical repertoire concerning nitrogen compounds. In essence, plant cells can recycle nitrogen-containing compounds more or less indefinitely. Consequently, because plants do not consume bulky foodstuffs the way animals do and generate no nitrogenous wastes, plants have no real excretory system. They also lack any specialized cells directly comparable to the muscles and nerves of animals. A few plants (e.g., the Venus flytrap) are capable of generating nerve-like action potentials that result in rapid leaf movements, but the resemblance to nerves and muscles is only rudimentary. There are no synapses, and rapid leaf movements are always driven by abrupt changes in turgor pressure (intracellular water pressure), not by contraction of muscle fibers.
The complementary nature of gas exchange in plants and animals is widely appreciated. Plants use carbon dioxide as a raw material for photosynthesis, yielding oxygen as a byproduct. Animals inhale oxygen and use it to break down food molecules to yield energy (via cell respiration), releasing carbon dioxide as a byproduct. It is somewhat less well-known that plant cells also perform a nearly identical process of cell respiration — burning food molecules, consuming oxygen, and releasing carbon dioxide. A healthy and actively growing plant during the daytime, however, does more photosynthesis than cell respiration and therefore releases more oxygen than it consumes. At night, of course, there is no photosynthesis, and cell respiration, with its “animal-like” gas exchange process, predominates.
Photosynthetic algae, though technically not plants, are biochemically similar. Hence the paradoxical situation of photosynthetic algae being responsible for depletion of oxygen from the water column can be readily understood, especially when undergoing a population explosion known as an algal bloom. During such conditions, all the algal cells are consuming oxygen at night, and the masses of dead and dying cells and the microbes that are busy decomposing them are consuming oxygen all the time.
Growth
Plants grow by means of special structures called meristems. A meristem is a group or layer of cells that has a long-term capacity to undergo the process of cell division; thus, meristems add new cells to the body of the plant. As new cells are made in the meristem, some remain in the meristem region and continue to divide, while others cease division, undergo cell enlargement, and become mature cells of the plant body. It is the nature of plant growth that new cells (organized as stems, leaves, or roots) are continuously added to the plant body.
Most plants have meristems located at the tips of stems (stem apical meristems) and at the tips of roots (root apical meristems) (fig. 12). These apical meristems are responsible for primary growth — the elongation of roots and shoots. Simply put, primary growth allows roots to extend through the soil and for shoots to grow upward, forming new leaves as they go, and at the appropriate time, also forming flowers (or other reproductive structures). Completely herbaceous plants possess only primary growth; all their growth is the result of the activity of apical meristems. Primary growth may be characterized as growth in length or extension growth.
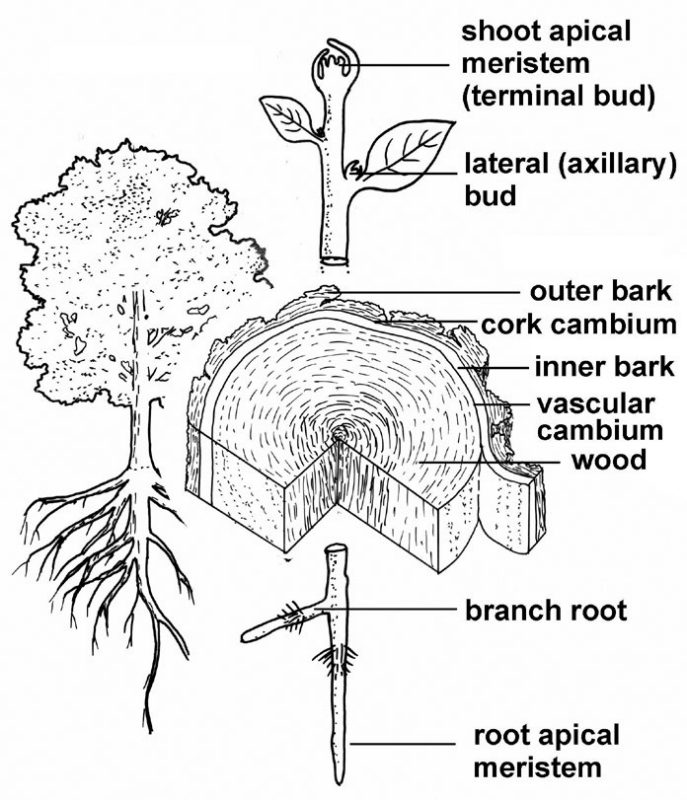
In addition to primary growth, woody plants also experience secondary growth, a process that results in increased thickness of plant parts. Two additional meristems are involved in secondary growth: the vascular cambium and the cork cambium (fig. 12). Whereas apical meristems are groups or masses of cells located at the tips of the plant, the cambia involved in secondary growth are cylindrical structures, one cell thick, embedded within the tissues of stems and roots.
Despite their structural differences, basic aspects of meristem function are the same for apical meristems and cambia: both have initials capable of repeated cell division that yield some derivatives that become part of the mature plant body. In terms of a general overview, secondary growth by the vascular cambium of woody plants produces wood and the inner or living bark; the cork cambium produces outer bark (fig. 12).
Hormones
Many details of plant growth are regulated at the biochemical level by molecules known as hormones. Typically, a hormone is a molecule that is present in small quantities but exerts large, easily observed effects as it influences the pattern of growth. For example, many plants experience a springtime surge in the production of auxin, a hormone with multiple influences on growth and development. This auxin surge stimulates the activity of plant meristems, thus initiating a new season of growth.
During primary growth, stems and roots will respond to various aspects of the environment, such as light, gravity, and touch, in processes known as tropisms that are also modulated by auxin hormones. In general, a tropism is a growth response that is oriented relative to an environmental stimulus. For example, stems typically grow toward bright sources of light, so they are said to be positively phototropic. Phototropism works because excess amounts of auxin accumulate on the shaded side of a stem, causing cells on the shaded side to elongate more than those on the sunny side, and resulting in a gradual bending of the stem in the direction of the light.
Plants respond to gravity in a generally similar fashion. Shoots are negatively gravitropic (they grow up) because relatively large quantities of auxin accumulate on the lower sides of horizontally oriented stems, causing those cells to elongate greatly and bending the shoot tip to a vertical orientation. Roots are typically positively gravitropic (they grow down), but the process seems to be a little more complicated; auxin is involved, but other hormones seem to play a role, too.
Another common tropism is thigmotropism, a growth response to touch or, more fundamentally, pressure. Thigmotropism is best illustrated in coiling tendrils and twining vines. When a tendril or stem of a vine contacts an object, the physical pressure at the point of contact eventually results in increased auxin concentrations in the cells opposite the point of contact. As those cells respond by greater elongation, it causes the tendril or vine to curl around the object.
In many plants, auxin also plays a role in determining whether lateral (axillary) buds will remain dormant or whether they will grow and become stem branches. In general, active apical meristems are sources of auxin, that then flows from cell to cell toward the base of the plant. In many plants, an intact apical meristem and strong downward flow of auxin molecules through the stem serve to inhibit growth of lateral buds. If, however, the apex is damaged or removed entirely, the interruption of the auxin flow stimulates development of lateral buds and, hence, branching. In essence, one or more new side branches replace the injured or missing original apex. Some plants, however, will branch spontaneously even in the presence of an intact meristem on the main stem.
In addition to auxin, there are several other plant hormones involved in the regulation of plant growth and development. Gibberellic acid is involved in the bolting of stems that characterizes the transition to flowering in many biennials that have a first-year rosette growth habit. Gibberellic acid also controls the transition from juvenile to adult phases of growth, as seen in English ivy (juveniles climb by adventitious roots, seldom branch, and have palmately lobed leaves; whereas adults cease climbing, branch profusely, have unlobed leaves, and make flowers and fruits). Ethylene and abscissic acid are involved in controlling leaf drop in autumn and other aspects of preparation for winter dormancy. More detailed information on plant hormones can be found in plant physiology textbooks.
Nutrients
Plant growth is fueled by the coordinated interaction of multiple factors, the most important of which include uptake of water and mineral nutrients from the soil and the photosynthetic production of sugars from sunlight and carbon dioxide from the air. Water and dissolved minerals are absorbed from the soil by roots and transported through the plant by the water-conducting system or xylem tissue. The three most important nutrient elements include:
- Nitrogen (N) – Essential for building proteins, nucleic acids like DNA, and energy-processing molecules.
- Phosphorus (P) – Also essential for nucleic acids and energy-processing molecules.
- Potassium (K) – Important in many enzymatic reactions and for maintenance of proper ionic balance in cells.
The importance of these three elements — N, P, and K — is illustrated by the fact that they are the components of commercial plant fertilizers.
Other important nutrients are:
- Calcium (Ca) – A necessary component of plant cell walls.
- Iron (Fe) – Essential in energy-processing molecules and for chlorophyll synthesis.
- Magnesium (Mg) – A component of the chlorophyll molecule.
Though also essential, micronutrients required in small amounts by plants include: boron (B), manganese (Mn), nickel (Ni), copper (Cu), zinc (Zn), molybdenum (Mo), and chlorine (Cl).
When present in appropriate amounts, plants generally can absorb mineral nutrients directly from the soil. However, it is not unusual for some elements to be scarce in the soil, and certain plants have developed symbiotic relationships with various microbes to assist in nutrient procurement. For example, legumes and a few other plants harbor bacteria that are capable of performing nitrogen fixation — the conversion of nitrogen gas from the air into ammonia. Whereas nitrogen gas is completely inert to plant and animal cells, ammonia is readily incorporated into other organic molecules. In general, symbiotic nitrogen fixation involves the plant feeding sugars to the bacterium and providing an oxygen-free space for the bacterium to live. In return, the bacterium allows the plant to absorb some of the ammonia it produces. In the case of legumes, nitrogen-fixing bacteria live in tight little knots of cells known as root nodules.
Mycorrhizae represent another widespread nutrient-procurement symbiosis. Mycorrhizae, literally “fungus roots,” represent close associations of plant root and fungal cells. Again, the plant feeds the fungus with sugars produced via photosynthesis. In return, the fungus provides the plant with a wide range of mineral nutrients that it obtains from the decomposition of dead organic matter elsewhere in the soil. Mycorrhizal associations are extremely widespread.
Transport via Phloem and Xylem
Regardless of how the soil-derived mineral elements are procured, green cells of the shoot system (i.e., certain stem and leaf cells) incorporate these minerals into the myriad organic compounds of plant cells; these organic compounds are derived ultimately, of course, from the carbon backbone of sugar molecules made via photosynthesis. Excess sugars and other organic compounds are transported via phloem tissue from mature green cells of the shoot system to the root system and/or to any young (i.e., actively growing) part of the shoot system.
In general terms, phloem transport occurs from “source to sink,” i.e., from regions where sugars are present in abundance to areas where sugars are being consumed, either to build the walls and cytoplasm of new cells or to be consumed for energy purposes. To a large extent, what constitutes a source and what constitutes a sink vary with the season and physiological activities of the plant. In early spring, for example, stored food located in roots, bulbs, corms, etc., constitute the source, and the rapidly growing (or regrowing) shoot system is, temporarily, a sink.
Xylem, the water-conducting tissue of vascular plants, performs important multiple functions. Water obtained from the soil and transported to the stems and leaves is critical for routine functioning of the plant. The process of photosynthesis requires entry of carbon dioxide gas from the atmosphere into the plant via microscopic pores called stomates. When stomates are open, carbon dioxide diffuses in and water vapor diffuses out. In essence, the plant “spends” water obtained from the soil and transported to photosynthetic tissues in order to “buy” carbon dioxide necessary for photosynthesis.
As mentioned previously, mineral nutrients from the soil dissolve in soil water and enter the plant as part of the water transport process. Most of the water taken up by the plant evaporates away, leaving the dissolved mineral nutrients that become incorporated into the cell walls and living cytoplasm of the plant body via various biochemical pathways.
Finally, xylem tissue is composed of tough, durable cells. Their cell walls contain appreciable amounts of lignin, rendering them hard and physically strong. Another function of xylem, then, is provision of a strong structural framework inside the plant body. Wood is xylem, and its role in providing mechanical strength to trees is obvious. However, much smaller strands of xylem penetrate the finest twigs, stems, and leaves where their structural properties are no less important.
Flowering
Growth and development in plants is often coordinated with environmental signals so that phases of active growth, reproduction, and dormancy occur at the appropriate season. The most important environmental signals are seasonal fluctuations in temperature and the relative length of daylight and night, also known as photoperiodism. Many plants use the seasonal changes in photoperiod as a cue to initiate flowering. Long-day plants flower when the photoperiod (daylight hours) exceeds some genetically determined threshold value; typically, long-day plants in Virginia flower in the summer. Short-day plants flower when the photoperiod (daylight hours) is less than some genetically determined threshold value. In Virginia, short-day plants may flower in the spring or fall. In so-called day-neutral plants, flowering is not influenced by photoperiod; these plants may flower in any season once they are sufficiently mature and if the weather permits. The mechanism of flower induction by photoperiod is complex and involves a light-sensing pigment called phytochrome, an internal biological clock, and a flowering-inducing hormone (florigen) made in the leaves that travels to the apical meristems and converts them from production of vegetative tissues to formation of flowers.
Dormancy
Successful flowering results in the formation of a crop of seeds. In the temperate zone, many seeds mature and disperse with the embryo in a dormant condition. Seed dormancy may be controlled by a variety of factors, either acting alone or in combination. Dormancy is often imposed merely by desiccation, and rehydration of the seed is sufficient to initiate germination. A tough seed coat may assist dormancy by slowing water absorption, by slowing diffusion of oxygen into the seed, or merely by the physical restraint imposed by its tough structure. Physical wearing away or microbial decomposition of tough seed coats will often promote seed germination.
In some cases, the presence of chemical compounds enforces dormancy, and germination is possible only when the concentration of the inhibitory chemical diminishes to a certain point. In other cases, release from seed dormancy requires exposure to cold temperatures followed by return of temperatures favorable for growth. Because release from dormancy can be slow, viable but dormant seeds can accumulate in the soil and constitute what is known as a seed bank. The longevity of seeds in soil seed banks varies tremendously with the type of plant and the physical conditions of the soil. Certainly, survival for many years is possible. Dormant seeds of the soil seed bank can often be induced to germinate by a drastic alteration of the environment, for example following a forest fire.
Toward the end of the growing season, many plants undergo processes to prepare for dormancy through the winter season. For example, food reserves are transported to the root system or to underground stems for safe storage. Both primary and secondary growth stops and shoot apical meristems become covered with protective bud scales. Depending on the species, bud scales can be modified stipules or modified leaves with a hard, protective texture and waxy, water-retaining characteristics.
Finally, many plants undergo leaf drop or abscission as one strategy for surviving via dormancy. Many dormancy-inducing phenomena are controlled by the plant hormones ethylene and/or abscissic acid. In the case of leaf abscission, auxin also plays a role. Vigorous, healthy leaves generate an auxin supply that inhibits leaf abscission; as the plant enters dormancy, auxin production wanes and the effects of ethylene and/or abscissic acid predominate. This shift in hormones causes changes in a zone of cells (abscission zone) located at the point of petiole attachment to the stem. These cells become weak and eventually the leaf falls away, leaving behind another layer of newly exposed cells, the leaf scar. Plants that drop their leaves in the autumn are called deciduous. Evergreen plants retain their leaves through the winter; nevertheless, their level of biochemical activity is greatly diminished during periods of low temperature, so they, too, can be considered at least somewhat winter dormant.
Adaptations of Plants to Environmental Factors
Water
Among the myriad forms of plants are a number of common themes that illustrate adaptations to environmental factors. For example, availability of water varies tremendously across the environment, and different plants have various strategies that permit survival in conditions ranging from very wet to very dry.
Many familiar terrestrial plants live under conditions of moderate moisture availability where soils often contain sufficient water to support plant life. Moderate moisture availability is known as mesic conditions, and plants found under mesic conditions are known as mesophytes. Most terrestrial habitats in Virginia can be considered mesic. While mesophytes often have adequate water supplies, they still have adaptations to conserve water and to survive some periods of drought stress. Thus, mesophytes have moderately thick cuticle layers covering all surface cells exposed to the environment; the waxy cuticle helps these cells retain water. Mesophytes have ordinary stomata (surface pores) that function in the usual way, i.e., they are only open during daylight hours when there is sufficient water available to permit some to escape from the leaves while carbon dioxide needed for photosynthesis diffuses inward. (Again, it is useful to imagine plants “spending” water in order to “buy” carbon dioxide.)
Environments that experience frequent dry conditions are described as xeric, and plants adapted to xeric environments are known as xerophytes. A desert would be a familiar example of a xeric environment. In Virginia, xeric environments are encountered where soil conditions are such that rainwater quickly evaporates or runs off, resulting in stressfully dry conditions. Common xeric environments include rocky cliffs and ledges with small bits of soil in pockets or crevices, talus slopes composed of loose stones and gravel, and coastal sand dunes. Xerophytes exhibit various water-conserving adaptations. Cuticles tend to be very thick. Stomata may be sunken below the leaf surface and/or covered with long hairs to slow the rate of water loss. Leaves and/or stems are often thick and succulent from the existence of water-storing tissues.
Many succulent plants have a physiological syndrome known as Crassulacean acid metabolism (CAM). In CAM plants, stomates open at night when carbon dioxide can diffuse inward with relatively low amounts of water loss. Of course there is no light for photosynthesis at night, so the carbon dioxide is stored temporarily as acid molecules that accumulate in the succulent water-storing tissue. During daylight hours, the stomates remain closed and carbon dioxide for photosynthesis is provided by the breakdown of the stored acids.
Another physiological adaptation common in xerophytes (but also found in mesophytes) is C4 photosynthesis. C4 plants have a unique pattern of chlorophyll-bearing cells clustered around the leaf veins. In addition, there are some biochemical adaptations that allow for efficient photosynthesis in C4 plants when it would be too hot and too dry for ordinary plants to be productive.
Wetland environments may be characterized as hydric and are populated by hydrophytes. Wetland plants usually have plenty of water to support ordinary photosynthetic physiology; cuticle layers tend to be thin, and stomates tend to be plentiful and well-exposed. However, the presence of abundant water provides other challenges. For example, waterlogged soils often lack oxygen, yet the cells of plant roots require oxygen to stay alive. In many wetland plants, there are internal aerenchyma tissues riddled with air pockets and passageways that serve as a sort of internal ventilation system permitting diffusion of oxygen into the roots. In some hydrophytes, e.g., water lilies, airflows from mature leaves down to the stems located in the mud and back out the young leaves — all through aerenchyma tissue.
Pollination
The diverse forms of flowers often prove to be adaptations to facilitate pollination.
- Wind-pollinated flowers tend to be stripped to the essentials: pollen-bearing stamens, often produced in abundance, and large, feathery, pollen-catching stigmas. Floral visitors are not needed, so both showy petals and nectar-producing structures are absent.
- Flowers pollinated by animals typically have attractive features that serve to catch the animal’s attention and orient its behavior to the flower. Attractive features may be visual, such as bright, nongreen petals, or they may be chemical fragrances wafted into the air.
- Bees see well in the blue and ultraviolet end of the light spectrum. Consequently, the petals of bee-pollinated flowers are often shades of blue, purple, or violet, and they often bear patterns of lines or spots called nectar guides that direct the bee to the center of the flower. These flowers reward the bees by providing nectar and excess pollen that the bees collect to support their growth. Many bee-pollinated flowers will release a shower of pollen in response to the bee’s “buzz” behavior as it pushes into the flower to reach the nectar.
- Hummingbirds see well in the red end of the light spectrum, and red is a common color found in many hummingbird-pollinated flowers. Hummingbirds have very high metabolic rates, and hummingbird-pollinated flowers provide abundant sweet nectar as their pollinator reward. The nectar is often located near the bottom of long, tubular corollas that the hummingbird can reach via its long beak and tongue. Stamens and stigmas are typically oriented to brush against the hummingbird’s head in order to bring about pollen transfer.
- Butterfly-adapted flowers are similar to those pollinated by hummingbirds in terms of their petal color and their nectar production and location, which the butterflies reach via their long, coiled mouthparts.
- Moths have mouthparts similar to butterflies, so moth-pollinated flowers also tend to be tubular and produce nectar rewards. Because most moths are active at night, moth-pollinated flowers tend to have pale or white petals that show up in dim light, and they often emit fragrances that the moths can detect from long distances.
Seed Dispersal
In seed plants, pollination leads directly to the formation of a crop of seeds contained in cones of conifers or fruits of angiosperms. In some plants, there is scarcely any dispersal of seeds; they merely drop passively from the parent plants. Many plants, however, possess adaptations that promote the dispersal of these seeds away from the parent plant — a strategy that reduces competition among the seedlings and between the parental and seedling generations.
Small, lightweight seeds can be dispersed effectively by the wind. The dust-like seeds of orchids are a good example of wind-based seed dispersal. Structural adaptations like plumes and bristles of dandelions and thistles can enhance buoyancy of seeds in air and thus promote their dispersal across the landscape. Similarly, wing-like extensions of fruits, as in ash (Fraxinus spp.) or tree-of-heaven (Ailanthus altissima), or wing-like extensions of half-fruits, as in maples (Acer spp.), represent additional adaptations for wind-assisted dispersal.
A few plants have explosive fruits that disperse their seeds in a ballistic fashion. Examples include the exploding dry capsules of spurges (Euphorbia spp.) and members of the acanthus family or the fleshy fruits of cucurbits, which explode from the build-up of fermentation gases produced by microbes.
A great many plants produce fleshy fruits that are attractive to animals and provide important nutrients when consumed. The seeds contained within these edible fruits often pass intact and viable through the gut of the animal, which is likely to defecate the seeds at some distance from their source. Other seeds or seed-containing fruits are covered with spines or bristles, often hooked or barbed at the tip, that are adapted for entanglement in fur, feathers, or clothing, allowing them to achieve dispersal by “hitchhiking.”
Taxonomy of Plants
Classifications of Plants Change Over Time
Major taxonomic categories of vascular plants are described previously. Because angiosperms (flowering plants) are so diverse, it is important to include an overview of how they are classified. There is no single classification of the angiosperms. Linnaeus published an early comprehensive classification of plants in his seminal work, Species Plantarum, in 1751. The Linnaean classification was based on counting flower parts. First, the number of stamens determined the general categories or “classes,” within which the number of carpels defined “subclasses.” The overall hierarchy in Linnaean classifications consisted of four levels: class, subclass, genus, and species. All subsequent classifications of angiosperms have been hierarchical, but it has been long recognized that defining taxonomic groups based on one or two characters (e.g., stamen and carpel counts) is artificial.
Numerous post-Linnaean classifications were proposed and most were intended to represent natural relationships that, since Darwin, have meant that taxonomic groups are intended to represent actual genetic descent in evolutionary terms. The German botanist Engler developed a post- Darwinian classification of flowering plants that can be characterized as progressive. The sequence of angiosperms started with those possessing simple flower structure (e.g., catkin-bearing trees) and culminated with plants bearing very complex flowers (e.g., daisies or orchids). Engler’s classification was comprehensive and global in scope and was widely adopted in its day (late-19th and early-20th centuries). Many floras and reference works published in the late-20th century still followed classifications deeply rooted in the Englerian system.
Late in the 20th century, however, several other botanists proposed equally comprehensive reclassifications of the flowering plants. Differing somewhat in detail, the classifications by Cronquist, Thorne, and Takhtajan all started with Magnolia (fig. 1) and its relatives as most similar to the first flowering plants, with other major groups hypothesized to diverge and diversify from that ancestral core. These taxonomists drew on diverse data sources in defining their taxonomic categories. They went far beyond traditional gross morphology, weighing the importance of microscopic anatomy, details of embryological development, pollen structure, chromosomes, and comparative biochemistry. Consequently, these late-20th-century classifications can be characterized as synthetic or integrative, but they are also intuitive in that the classifications essentially represented the botanical scholar’s mental model of the evolutionary connections between groups.
It should be remembered that a classification is a hypothesis about how the classified organisms are related, and it is in the nature of science that hypotheses are modified to reflect the discovery of new data. It should not be surprising, therefore, to realize that classifications have changed — sometimes rather drastically — with the passage of time and the advance of botanical study.
Starting with the foundations provided by earlier classifications of angiosperms, current (modern) classifications reflect the impact of two recent revolutions in taxonomy/ systematics. The first of these is the adoption of cladistic analyses. Briefly, cladistics provides a philosophical foundation for the rigorous reconstruction of evolutionary connections between organisms; moreover, computer programs have been developed to apply cladistic principles to very large data sets, yielding reproducible and statistically compelling models of relationships.
The second revolution is the incorporation of data derived from DNA (gene) sequence data. Simply put, there are many genes in plants, and each gene has the potential of revealing numerous taxonomically significant variants when sequences from numerous plants are compared.
The results of these ongoing revolutions in plant classification have been disseminated in a series of three landmark publications authored by a consortium of botanists who call themselves the Angiosperm Phylogeny Group. One important insight derived from these molecular phylogenetic studies is the recognition that flowering plants fall into three major groups:
- Basal angiosperms – A somewhat heterogeneous group of plants that represent the modern descendants of some very early branches of the evolutionary tree.
- Monocots – More or less as traditionally defined, plants with one cotyledon, scattered stem bundles, pollen with a single pore, and flower parts in threes.
- Eudicots – Plants with two cotyledons, stem bundles in a ring, pollen with (usually) three pores, and flower parts mostly in fours or fives.
Some Prominent Flowering Plant Families
One convenient way to assimilate flowering plant diversity is to begin by learning how to recognize several common and distinctive plant families. There are approximately 200 families of vascular plants found spontaneously in Virginia. Following are a few examples of prominent angiosperm (flowering plant) families.
- Poaceae – Grass family (fig. 13). Monocot herbs with round stems and leaves in two ranks; flowers minute and organized into spikelets containing one or more flowers; each spikelet enclosed at its base by two more-or-less similar bract-like glumes; each flower enclosed by a pair of dissimilar bracts — the palea and lemma.
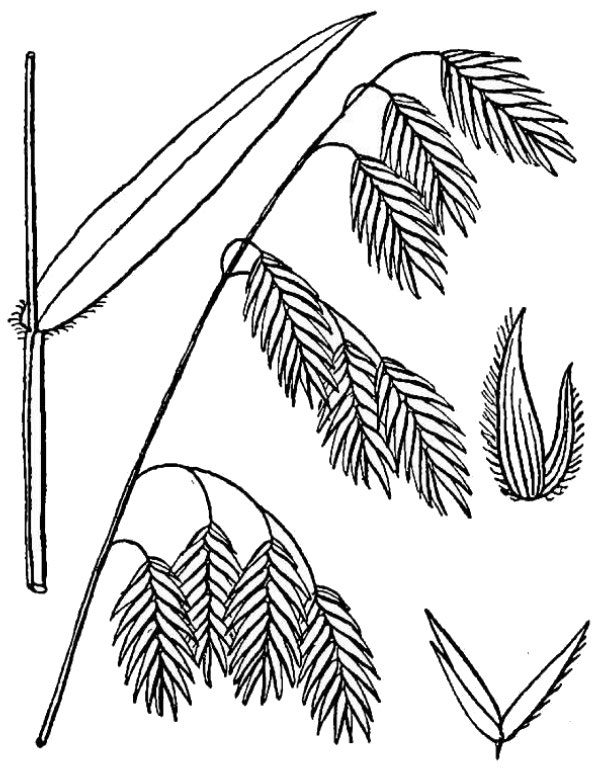
- Cyperaceae – Sedge family (fig. 14). Monocot herbs, often with triangular stems and leaves in three ranks; flowers minute and organized into spikelets; each reduced flower subtended by a scale-like bract.
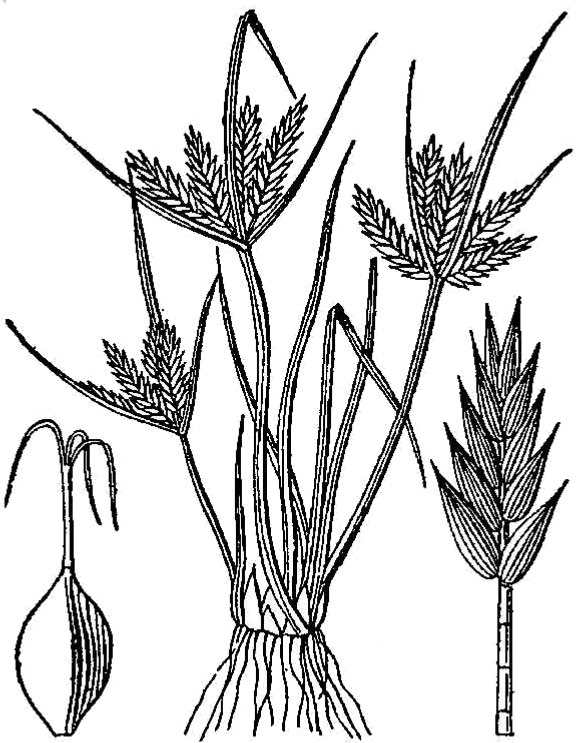
- Juncaceae – Rush family (fig. 15). Monocot herbs; flowers small, greenish, complete; three sepals; three petals; three or six stamens; pistil three-lobed; ovary superior; seeds numerous.
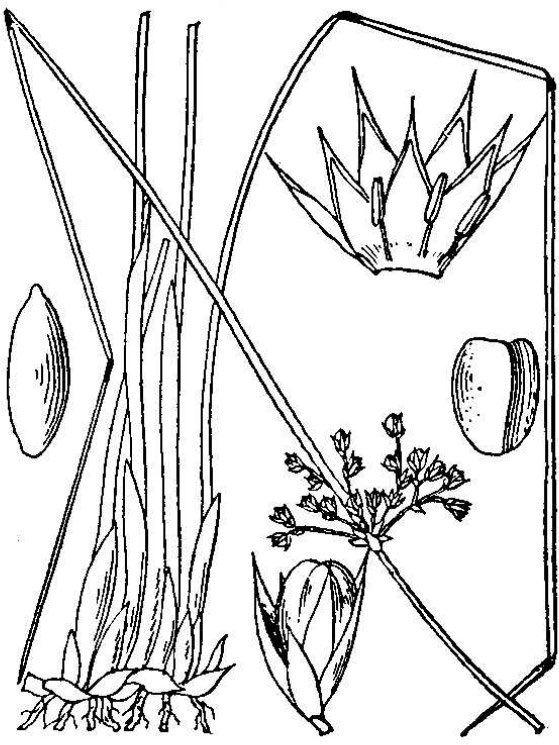
- Orchidaceae – Orchids (fig. 16). Monocot herbs, terrestrial in Virginia but often epiphytic in the tropics; flowers with three sepals and three petals, one of which specialized as a prominent “lip”; pollen shed in compact masses called pollinia; ovary inferior; seeds minute and dust-like.
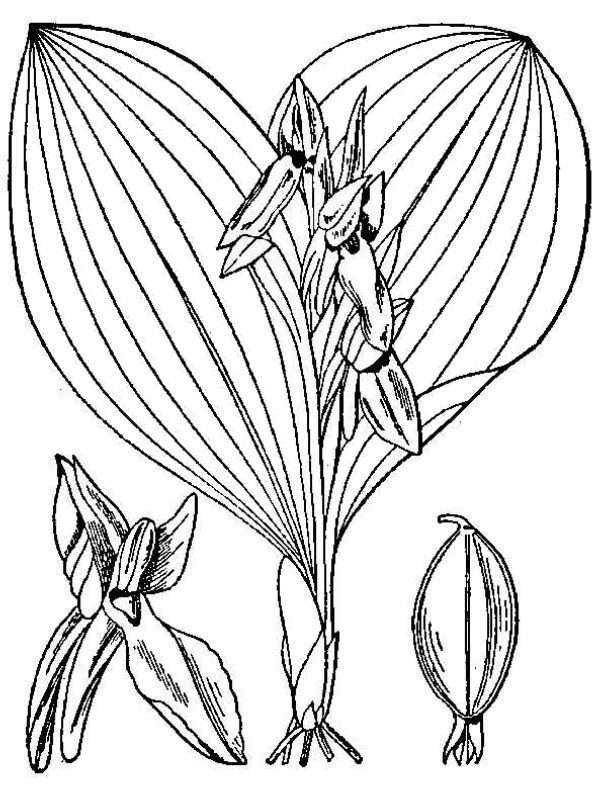
- Asteraceae – Daisy family (fig. 17). Eudicots, often shrubby or herbaceous; flowers small, grouped into daisy-like clusters; ovaries inferior, maturing into single-seeded fruits.
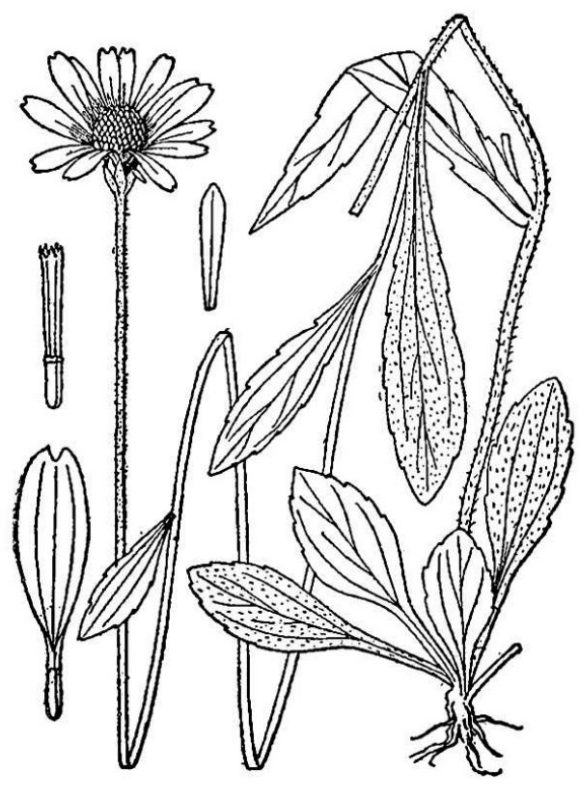
- Fabaceae – Legume family (fig. 18). Eudicots of various habits; roots bear nodules containing symbiotic nitrogen-fixing bacteria; leaves typically compound, with a hinge-like pulvinus at the base and below each leaflet; flowers pea-like, with one vertical “standard” petal, two lateral “wing” petals, and two forward-projecting “keel” petals that enclose the stamens and single carpel; fruit a dry pod.
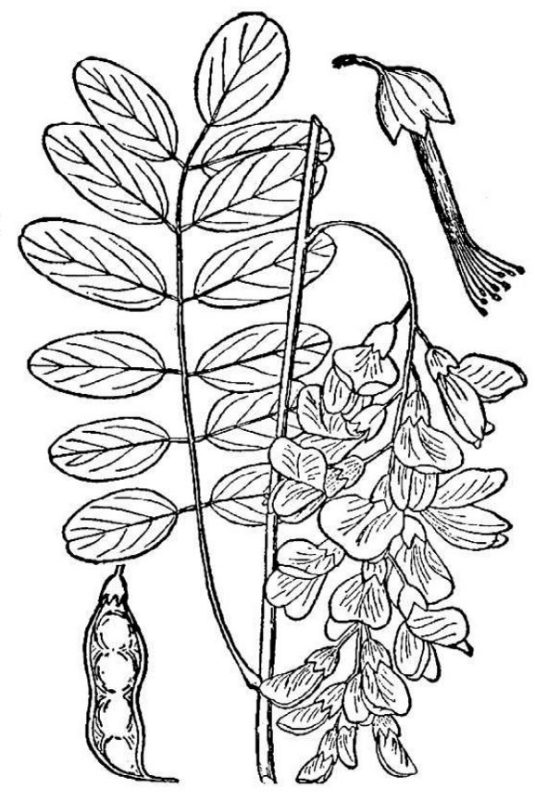
- Lamiaceae – Mint family (fig. 19). Fragrant eudicot herbs; stem often square in cross-section; leaves opposite; flowers usually arranged in whorled clusters; petals fused forming upper and lower “lips”; fruit consists of four small nutlets enclosed within the persistent sepals.

- Rubiaceae – Madder family (fig. 20). Eudicot herbs or shrubs (or trees in the tropics); leaves opposite, with stipules often extending between the petiole bases; flowers with fused petals; ovary inferior.
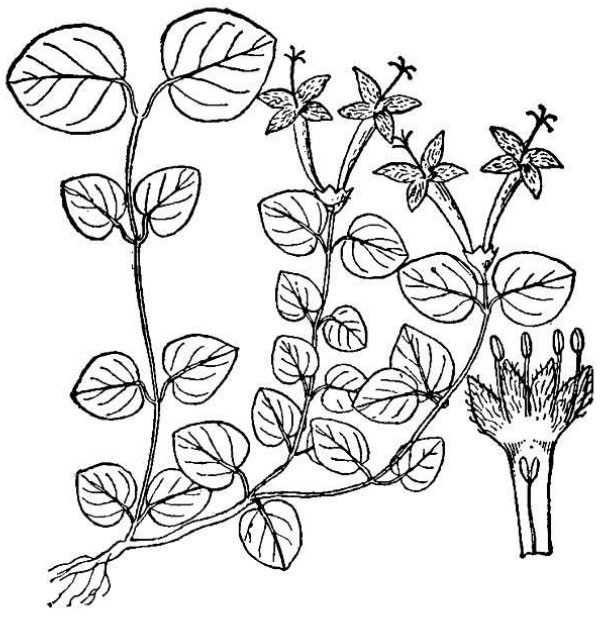
- Brassicaceae – Mustard family (fig. 21). Eudicot herbs containing pungent glucosinolate molecules; leaves alternate; flowers in flat-topped clusters that elongate into raceme-like patterns of fruits; four petals; six stamens, often of unequal length; ovary superior, consisting of two carpels; fruits dry, dehiscent.

- Apiaceae – Carrot family (fig. 22). Eudicot herbs, often aromatic; leaves often compound with a sheathing leaf base; flowers small, produced in simple or compound umbels (flat-topped clusters); ovary inferior; fruit splitting in two halves, each containing a single seed.

- Rosaceae – Rose family (fig. 23). Eudicot herbs, shrubs, or trees; leaves alternate, toothed, either simple or compound, associated with stipules at the base; flowers with separate petals and many stamens; ovary and fruit highly variable.

Key Characters Used to Identify Plants
Habit
The overall growth habit of plants can vary tremendously from the minute green blobs that constitute duckweeds floating on a pond to towering forest trees. A few basic categories are fundamental.
- Herbs (adjective: herbaceous) are plants that produce very little woody tissue in their stems and/or produce aboveground stems that persist for just a single growing season.
- Shrubs (adjective: frutescent) are smallish woody plants whose stems often grow in clumped fashion and are generally less than 25 feet tall.
- Trees (adjective: arborescent) are woody plants, usually single-stemmed and taller than 25 feet.
- Subshrubs (adjective: suffrutescent) are intermediate between herbs and shrubs.
- Lianas are plants with a vine-like habit. Lianas employ various growth modifications in order to grow on and over other vegetation. Some use grasping tendrils; others grow in a twisted pattern and twine around supporting structures; some use spines, thorns, prickles, or short spur shoots to snag the branches of other plants like grappling hooks; yet others merely grow rampantly over the canopies of other plants.
Duration
- Annuals complete their life cycle (seed to seed) in a single growing season.
- Biennials require two years, typically growing as a rosette — a cluster of leaves at groundlevel attached to a short stubby stem — the first year and then bolting the second year, producing a tall stem bearing flowers, fruits, and seeds.
- Perennials persist for many years. Herbaceous perennials often die back at the end of the growing season and survive the winter months as a dormant underground structure. These so-called rootstocks take various forms, many of which are actually modified stems.
- Rhizomes and tubers are horizontally oriented stems. Rhizomes are moderately thick and decidedly elongate (fig. 24). Tubers are very thick and fleshy, generally not much longer than they are wide (fig. 25).
- A corm is a vertically oriented underground stem in which the food is stored in stem tissue; it is often protected by papery scale-like leaves (fig. 26).
- A bulb is also a vertically oriented, underground stem, but in a bulb, the food is stored in a series of fleshy leaves (fig. 27).
- Stolons or runners are elongate, and are more or less leafless horizontal stems at the soil surface or at a shallow depth in the soil, the tips of which generate new plants (fig. 28).



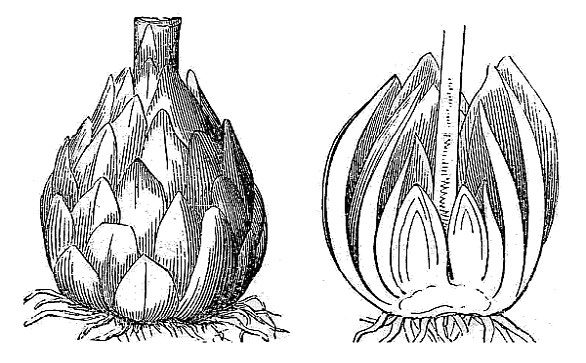
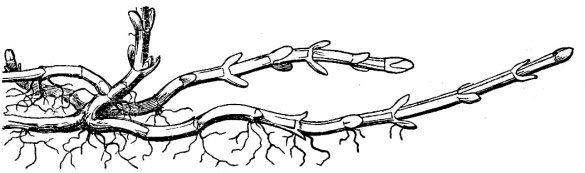
Root Systems
Although often out of sight and out of mind, root systems provide important clues for the identification of plants.
- A diffuse root system consists of numerous highly branched, more-or-less fine-textured roots, none of which is appreciably larger than the rest.
- A tap root system is dominated by a single large root modified for food storage.
In many plants, the root system is derived completely from the first root that emerges from a germinating seed. In other plants, additional adventitious roots arise from within stems (and less commonly, leaves). Adventitious roots may have specific functions indicated by descriptive names, for example, prop roots or climbing roots.
Leaves
Stems bear leaves. The portion of a stem to which a leaf is attached is known as a node; the intervening leafless portions of the stem constitute the internodes. Nodes can be very close together, resulting in a clustering of leaves, or the internodes can be elongate, resulting in well-separated leaves. Also, the number of leaves per node is often a useful character in plant identification. The basic patterns of leaf arrangement/attachment are:
- Alternate – One leaf per node (fig. 29).
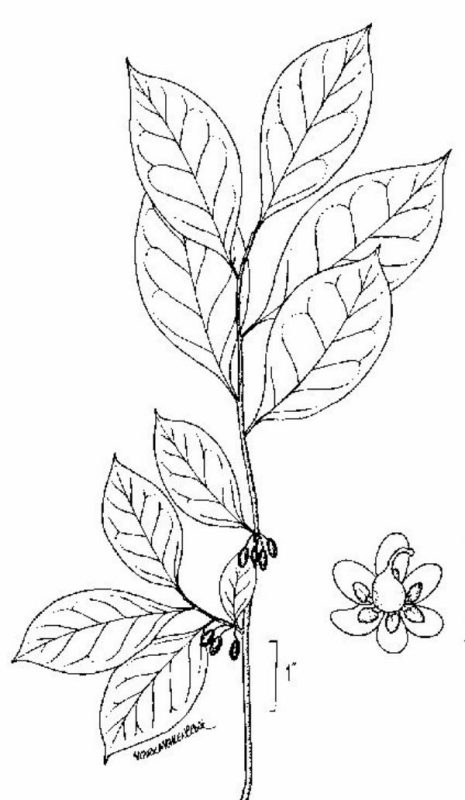
- Opposite – Two leaves per node, typically on opposite sides of the stem (fig. 30).
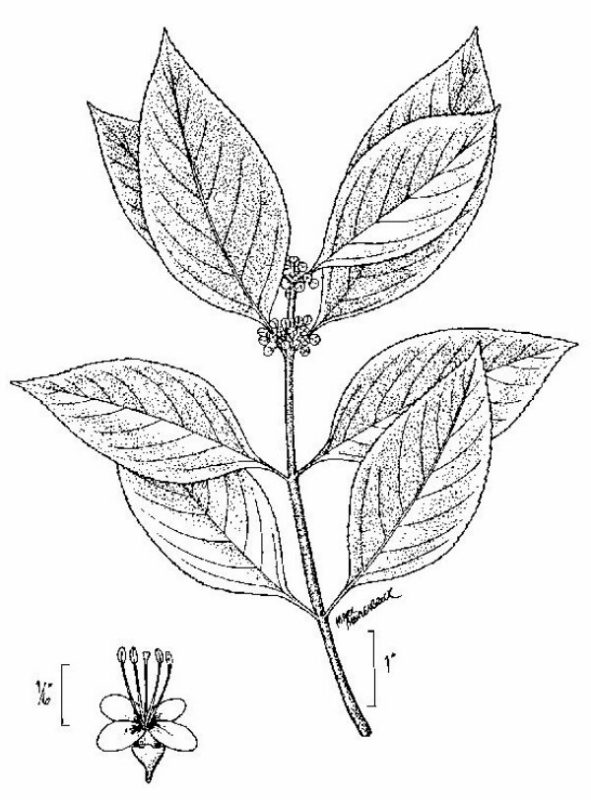
- Whorled (or verticillate) – Three or more leaves per node (fig. 31).

Leaves are typically organized into a broad, photosynthetic portion (leaf blade or lamina) and a more slender portion (petiole or leaf sheath) that connects the leaf to the stem. Leaves lacking petioles or sheathing bases are said to be sessile. In a simple leaf, the blade is an undivided single structure (figs. 29, 30, and 31). In a compound leaf, the blade is subdivided into multiple leaflets. The leaflets of compound leaves can occur in several different patterns, but it is useful to note that they generally are all oriented in the same flat plane.
Pinnately compound leaves (fig. 32) have leaflets attached laterally along the sides of an extension of the petiole known as the rachis. Pinnately compound leaves can be further distinguished as odd-pinnate (fig. 32A) or even-pinnate (fig. 32B), depending on the presence or absence of a terminal leaflet. They can also exhibit multiple levels of division. Thus, a once pinnately compound leaf merely has a series of single leaflets attached to the rachis (fig. 32A-B); a twice pinnately compound leaf has leaflets that are themselves subdivided into finer leaflets (fig. 32C).
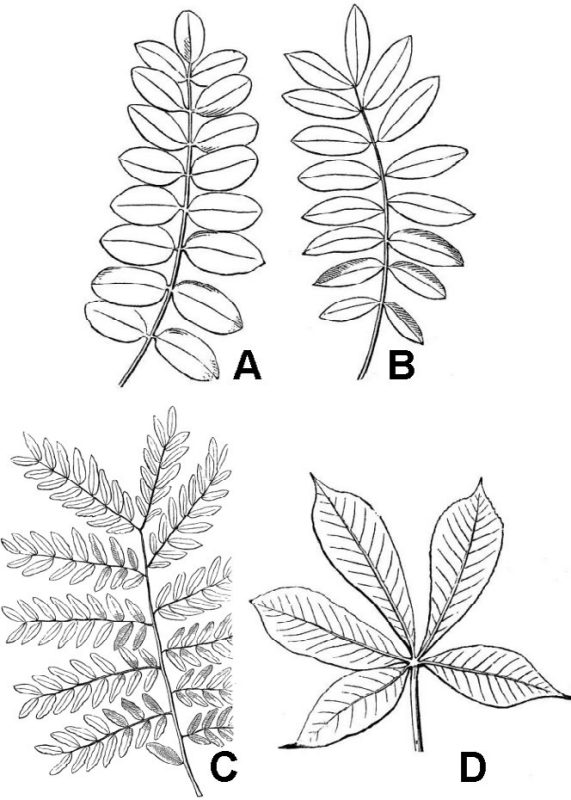
Palmately compound leaves have multiple leaflets that diverge from the apex of the petiole (fig. 32D). A compound leaf consisting of three leaflets is termed trifoliolate (fig. 33D). Trifoliolate leaves can be considered to be fundamentally pinnate or palmate, depending on the presence or absence of a short rachis between the terminal leaflet and the two laterals. Lobed leaves are morphologically intermediate between simple and compound leaves (fig. 33A-C). Leaf blade tissue is continuous between the lobes of a simple leaf but discontinuous between the leaflets of compound leaves.
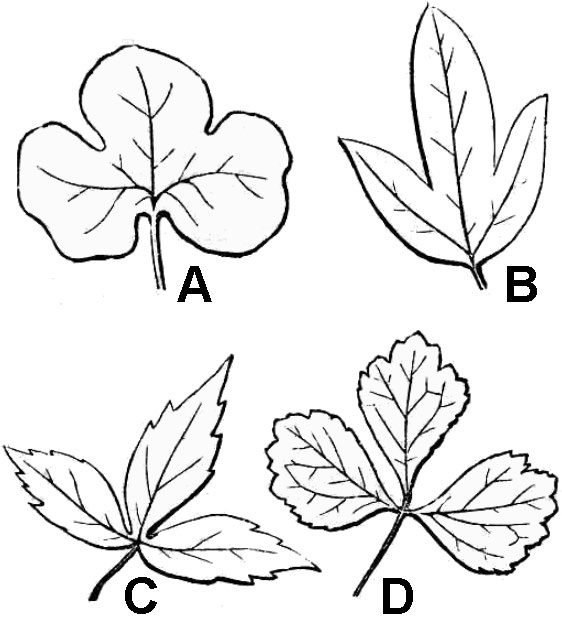
Many leaves are associated with flaps of tissue called stipules, usually located at the node or along the basal portion of the petiole. Stipules can assume many different forms. Though usually small, they can be large and resemble leaf blades; in other cases, they are modified into sharp-pointed spines, as in the black locust (Robinia pseudoacacia). They may function to cover young tissues at the stem tip, and they may persist on the plant or be shed as soon as its associated leaf is fully mature. Sometimes they are small and difficult to see without magnification. Plants lacking stipules are said to be estipulate.
The shape of leaf blades can vary tremendously among different plants and, to some extent, between different leaves on the same plant (figs. 34 and 35). Some commonly encountered terms for leaf blade shape include:
Linear – Narrowly elongate.
Oblong – More or less rectangular.
Elliptic – Elongate with symmetrically curved margins.
Ovate – Egg-shaped, with the widest portion below the middle.
Lanceolate – Elongate, widest at the base and tapered to a pointed tip.
Spatulate – Approximately spoon-shaped.
Reniform – Kidney-shaped.
The addition of the prefix “ob” denotes that the widest portion of the leaf is above the middle; thus we have obovate and oblanceolate. In a peltate leaf, the petiole inserts into the blade at some point other than the basal margin; in other words, blade tissue extends beyond the petiole tip.
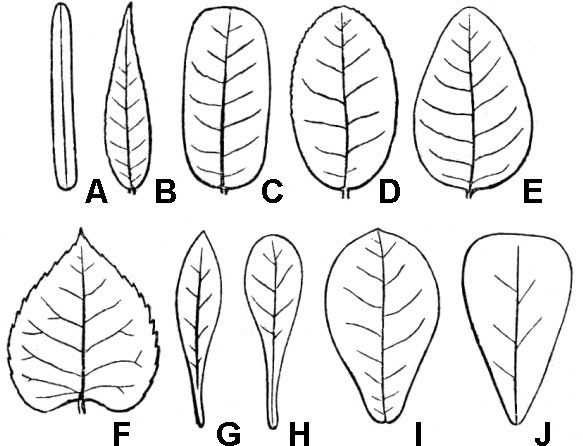
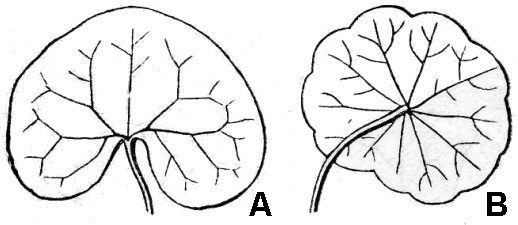
Leaf margins that display a relatively featureless, curved edge are said to be entire (fig. 34). Margins that curve downward are revolute. Margins cut into teeth can be serrate if the teeth project toward the apex (fig. 36A), or dentate if the teeth project straight outward (fig. 36B). Some plants have large, serrate teeth with finer teeth on the sides of each large tooth; such margins are known as doubly serrate.
Additional forms of leaf margin are illustrated in figure 36. There are many specialized terms to describe the geometry of leaf tips and leaf bases (where the blade joins the petiole). Some of these are self-explanatory; for example, rounded, acute, and obtuse. An acuminate tip tapers with a concave outline; retuse or emarginate tips have a shallow notch at the apex; an apiculate tip has a small, abrupt terminal tooth. A truncate leaf base appears to be cut off in a straight line.
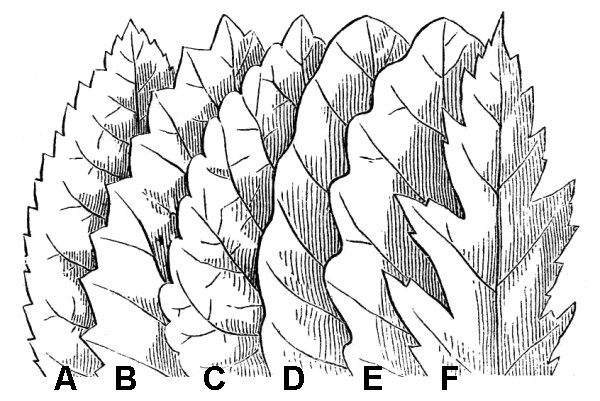
Inflorescence
An inflorescence is the pattern of flower production by a plant. The simplest inflorescence is a solitary flower. Solitary flowers are produced in two different ways, either at the end of the main stem (terminal; fig. 37A) or in the angle between a stem and a leaf (axillary; fig. 37B). Often, plants produce multiple flowers in an inflorescence, and there are two major patterns of doing so.
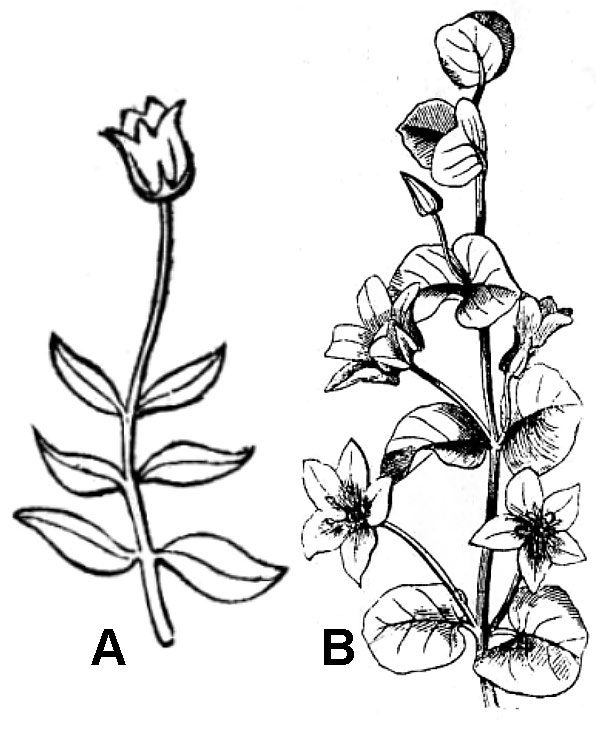
- Indeterminate inflorescences are characterized by the presence of a growing tip (apical meristem) that continues to make flower-bearing nodes of the inflorescence for an indefinitely long period of time. They can be recognized as flowering from the base to the tip, with older structures (flowers, fruits) toward the base and younger structures (flower buds) toward the tip. Two basic forms of indeterminate inflorescence are the spike, in which flowers are sessile on the inflorescence axis, and the raceme (fig. 38A), in which flowers are borne on pedicels (short, flower-bearing stems). A corymb (fig. 38B) is like a raceme but is special in that the pedicels and internodes elongate progressively so that the flowering region is flat-topped, and the developing fruits are well-separated along the sides of the inflorescence axis.
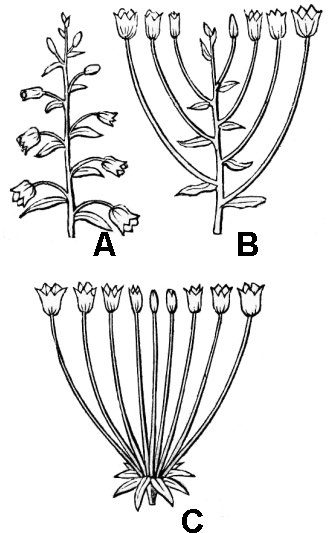
- Determinate inflorescences are characterized by a terminal flower that develops first, followed by additional flowers arising from nodes below. The simplest determinate inflorescence is a cyme (or dichasium; fig. 39A) with three flowers, the central/terminal flower opening first, followed by the two laterals forming below the pedicel of the first. Compound cymes (fig. 39B) repeat this pattern through multiple iterations, with pairs of lateral flowers forming below each flower that develops, at least until the flowering period stops.
Plants often produce flowers in tightly clustered patterns. Many pedicellate flowers arising from a common point constitute an umbel (fig. 38C). A compound umbel (fig. 22) consists of multiple umbel units, the stems of which are all attached to a common point.
A head or capitulum (fig. 17) consists of a tight cluster of sessile flowers. Umbels and heads can be derived from either determinate or indeterminate ancestors. Determinate heads and umbels have their first flowers open at their centers, whereas indeterminate heads and umbels start flowering at the outside edge and progress toward the center. Some highly modified cymes take on a coiled structure somewhat like the tail of a scorpion; often these special inflorescences are called helicoid or scorpioid cymes (fig. 40).
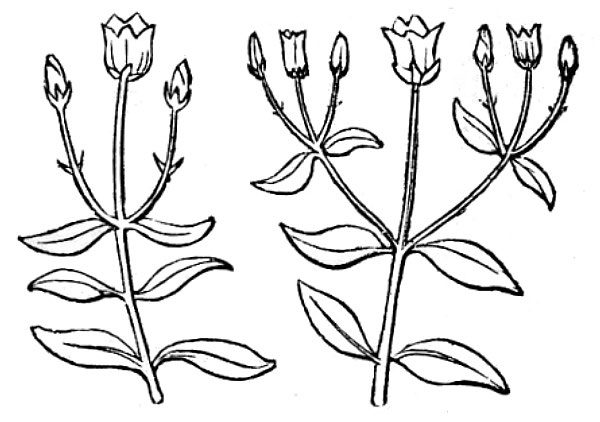

Special terms apply to profusely branched inflorescences.
- A panicle is an indeterminate inflorescence composed of multiple indeterminate branches, for example, a raceme-like mass of individual racemes or spikes.
- A thyrse consists of an indeterminate axis bearing lateral cymes.
- A catkin or ament (fig. 41) is a specialized inflorescence in which many highly reduced or simple flowers are organized in an elongate group; typically, each flower is unisexual, associated with one or more bracts and devoid of sepals and petals. A catkin or ament may, in its fundamental construction, be a spike, raceme, or thyrse, but in this case, these distinctions are considered secondary to the overall aspect of the inflorescence.
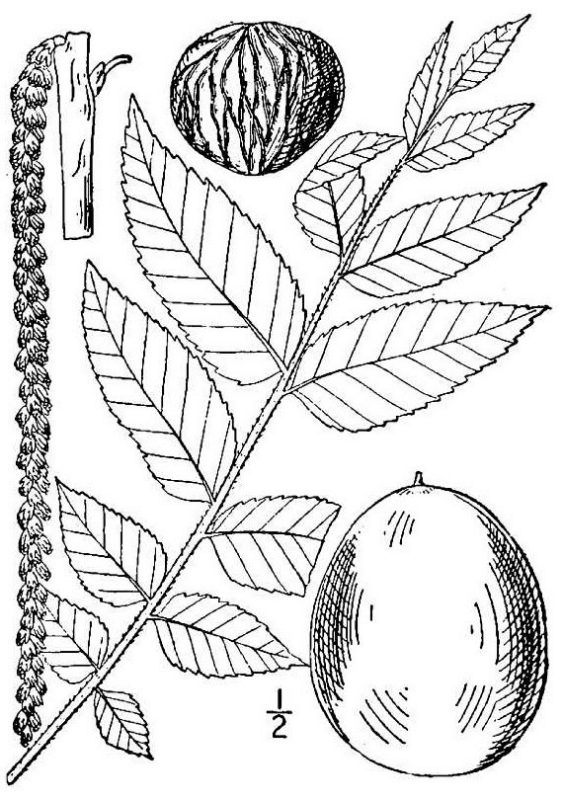
Flowers
Flowers occur in myriad forms. Potentially, each flower possesses four floral organs attached to the end (receptacle) of a flower-bearing stem or pedicel. When all four organs are present, they occur in a stereotypical sequence (fig. 42).
- The sepals are attached lowermost on the pedicel, and therefore, occur outermost in the flower bud that they serve to protect. Sepals are often green and quite leaf-like in appearance, but in many plants, they can also be brightly colored. Collectively, all the sepals of a flower constitute the calyx.
- Petals are next, and they are typically brightly colored, serving to attract pollinators to the flower. The collective term for all petals in a single flower is corolla.
- Next are the stamens, consisting of a stalk-like filament tipped with an enlarged pollen-containing anther. Because the pollen grains will eventually release sperm cells, stamens are commonly considered “male,” and collectively, all the stamens constitute the androecium.
- The innermost floral organ is the pistil, which consists of three distinct regions: (1) a terminal stigma that functions to receive pollen grains, (2) a basal ovary that contains the ovules, and (3) usually an elongate style functioning to connect stigma and ovary.
Because each ovule contained within the ovary will eventually produce an egg, the pistil is commonly considered “female”; all the pistils of a flower constitute the gynoecium. Eventually, the ovary region of the pistil will mature to produce a fruit that will contain one or more seeds derived from the ovules after becoming transformed by the plant’s sexual reproductive process.

A flower that possesses all four floral organs is said to be complete (fig. 42). One or more floral organs may be absent, in which case the flower is said to be incomplete (fig. 43). Whether complete or incomplete, if the flower contains both stamens and pistils, it is said to be perfect (or bisexual; figures 42 and 43B).
Imperfect (or unisexual) flowers posses either stamens or pistils, not both. Thus, imperfect flowers are often referred to as either staminate (male) or pistillate (female; fig. 43A).
Some species that form unisexual flowers produce them on different parts of the same plant; such plants are termed monoecious (sexes separate, but contained on one plant). Other species with unisexual flowers are dioecious, producing staminate and pistillate flowers on different plants (sexes separate, contained on two plants).
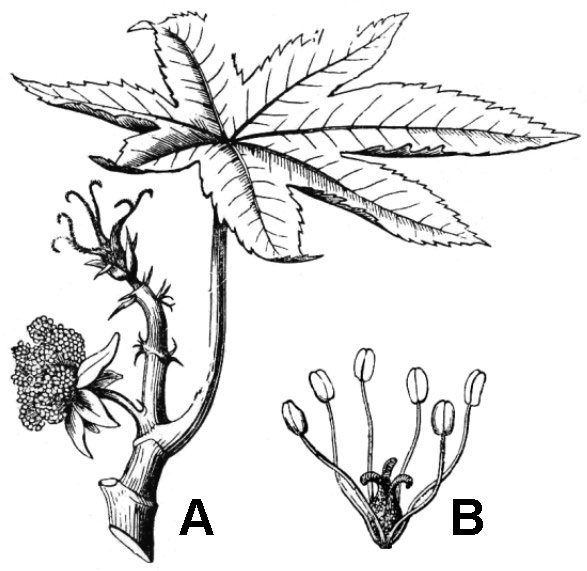
The numbers of floral organs per flower can vary tremendously among different species. It is common for the various floral organs to occur in multiples of small whole numbers; thus, one speaks of flowers that are 3-merous, 4-merous, or 5-merous. Other numbers can occur, and sometimes one of the floral organs can be present in very large numbers.
Floral organs of different species may occur in two basic patterns of symmetry:
- Radial symmetry (an actinomorphic flower) is characterized by symmetry about a central point, often visualized as the multiple pieces of a pie (fig. 44A).
- Bilateral symmetry (a zygomorphic flower) occurs as symmetrical halves or symmetry of reflection across a midline; like many familiar animals, such flowers have distinct right and left sides (fig. 44B).

Individual floral organs can be separate or fused with other organs of the same kind (connate fusion) or between different organs (adnate fusion). When petals are fused together, the corolla may assume a variety of 3-D configurations. Some corolla shapes may be described by self-explanatory terms like tubular and funneliform; other, less obvious formations, include campanulate (bell-shaped; fig. 45A), rotate (a flat disk, wheel-like shape; fig. 45B), or bilabiate (literally, two-lipped; figs. 43C, D, and E).
In flowers with fused corollas, it is often convenient to distinguish between the free extremities of the petals or the corolla limbs and their convergence at the throat. A nectar-containing, pocket-like extension of a single petal or portions of a fused corolla is termed a spur (fig. 45E). Stamens may be adnate to petals or connate (fused with each other; fig. 46). Connate stamens, with fused filaments forming a column-like structure with diverging anthers, are described as monadelphous (fig. 46B). Sometimes, sepals, petals, and the filament regions of stamens fuse together forming a tubular hypanthium.
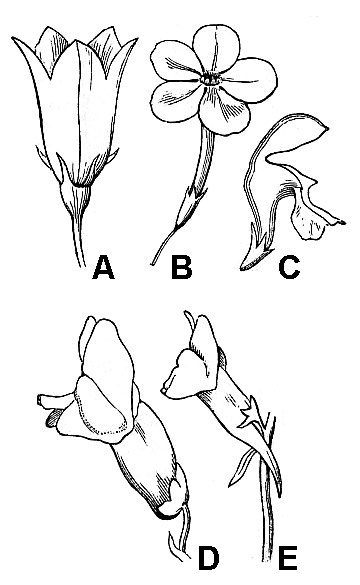
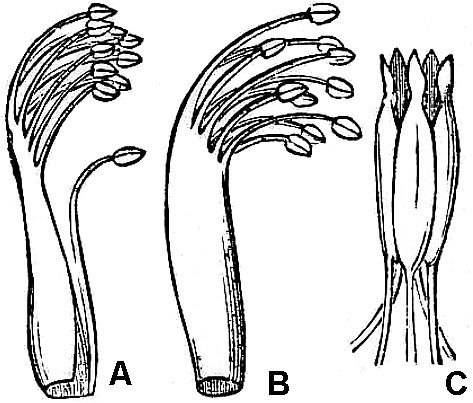
Organization of the pistils is highly variable among angiosperms (fig. 47). First, the number of pistils per flower can vary from one to an indefinite large number (multiple dozens). If there is more than one per flower, the pistils may be completely separate or they may be fused together to varying degrees.
Solitary pistils or completely separate multiple pistils are called simple pistils; each has its own separate and distinct stigma, style, and ovary. Compound pistils are composed of two or more pistils fused together in a single flower; lobes of their conjoined stigmas, styles, and/or ovaries indicate their origin from multiple pistils (fig. 47).
The term carpel is often used in reference to variations in gynoecium configuration. Most simply put, a carpel is a fundamental subunit of a gynoecium. Simple pistils consist of just a single carpel, and each lobe of a compound pistil represents a carpel unit.
One of the most significant themes of variation in gynoecium structure concerns ovary position relative to the point of divergence of other floral organs. A superior ovary (hypogynous flower) has sepals, petals, and stamens diverging below the ovary (fig. 48A). An inferior ovary (epigynous flower) has sepals, petals, and stamens diverging above the ovary (fig. 48D). Intermediate conditions, including ovaries surrounded by a hypanthium (fig. 48B) and ovaries that are half-inferior (fig. 48C) also occur.


Fruits
Typically, fruits mature either as dry or fleshy structures. Dry fruits may dehisce (open) spontaneously at maturity or they may remain indehiscent (unopened). Dehiscent dry fruits include the follicle (fig. 49A), a simple pistil that opens along a single suture; the legume (fig. 49B), a simple pistil that opens along two sutures; and the capsule (fig. 49C), a compound pistil that usually has multiple openings.
Indehiscent dry fruits usually contain a single seed and include the:
- Achene (fig. 49D) – The seed is loose within the fruit wall.
- Grain or caryopsis – The seed coat and fruit wall are tightly fused together (not illustrated, but an unpopped kernel of popcorn is a good example).
- Nut (fig. 49E) – Characterized by an extremely hard fruit wall.
- Nutlet – A small but very hard-walled fruit (not illustrated).
- Samara (fig. 49F) – A dry fruit with thin, wing-like outgrowths.
Fleshy fruits are termed drupes if they are one-seeded (fig. 50A, B) and berries if they contain multiple seeds (fig. 50C). In addition, apples, pears, and their relatives have pome fruits (fig. 50D), characterized by the presence of fleshy tissues in addition to those derived strictly from the ovary.


Simple fruits are the product of a single ovary of a single flower, whether that ovary consists of one or multiple carpels. The terms in the preceding paragraph refer to such simple fruits.
Aggregate fruits (fig. 51C) develop from multiple closely spaced but ultimately separate carpels that were produced by a single flower.
Multiple fruits (fig. 51D) develop from the ovaries of multiple closely clustered flowers. As with simple fruits, the individual units of aggregate or multiple fruits can mature as dry or fleshy elements.
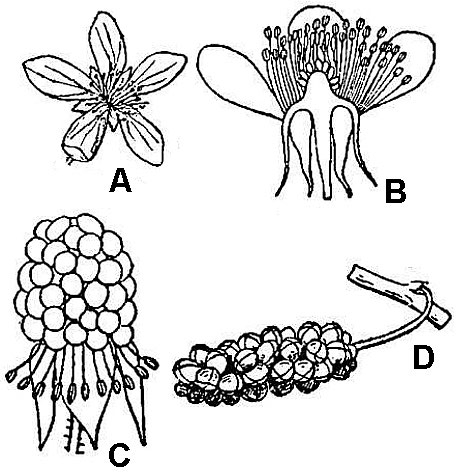
Threats to Plants in Virginia
Habitat
There can be little doubt that the single greatest threat to plants in Virginia is alteration of habitat — a process that is an inevitable consequence of human life. The current human population of the world and civilization as we know it came to exist because of the successful ways that we alter and exploit the habitats in which we live.
We live in houses and tend yards that seldom mirror local natural habitat. Our work and pursuit of recreation frequently take place in unnatural, man-made settings. We travel hither and yon, requiring roads, railroad tracks, and airports. To fuel our bodies, we farm vast acreages of land; to fuel our economy, we cut forests, dig mines, drill oil wells, and create large industrial complexes. We also need space to dump our trash.
Collectively, these activities alter natural environments and constitute our collective “human footprint.” Clearly, our presence on the planet comes at the expense of vast amounts of space that once functioned with little or no human impact. Our existence displaces plants and animals. Despite the extent of our footprint on nature, the future of many common species seems secure; natural habitats are still extensive, and many organisms can tolerate some degree of co-existence with people. However, rare species — simply because there are few individuals present and their geographic extent is limited — are especially vulnerable to habitat alteration by human activity.
Exotic Plants and Animals
One aspect of the human footprint requires special mention: our propensity to introduce organisms outside their natural range of occurrence. Any species present in an area outside its natural range can be considered exotic or alien. In the case of farming, horticulture, or the pet trade, the introduction of aliens is often intentional, but organisms can also be transported around the globe as unintended consequences of human travel and the trade and transport of merchandise and commodities.
While many exotic species can be considered beneficial, e.g., agricultural crops, others can “run wild” and impact native plants and animals in a wide variety of ways, chiefly by occupying habitat, i.e., taking up space that otherwise would be available to natives, and by altering the natural ecological function of that habitat. Exotic alien plants such as Japanese honeysuckle (Lonicera japonica), garlic mustard (Alliaria petiolata), kudzu (Pueraria lobata), Japanese stilt grass (Microstegium vimineum), Oriental bittersweet (Celastrus orbiculatus), and others are particularly threatening to native plants and animals because they actively and vigorously invade natural habitats. These species are often called invasive exotics.
Exotic animals can also threaten plant diversity. For example, the emerald ash borer, an insect, threatens serious impact on stands of our native ash trees. Depredation of our forest trees by the imported gypsy moth is another well-known example. Threatening exotics can also include pathogens such as the fungi that cause Dutch elm disease, chestnut blight, and dogwood anthracnose.
Overcollection
Another important threat to certain native plant species comes directly from humans: overcollection. Because most organisms have a reproductive capacity greater than what the environment can support, it should, in theory, be possible to collect or remove some number of individuals from nature with minimal impact on the long-term sustainability of the population. However, if demand is sufficiently great or the financial incentive is sufficiently strong, people can and do collect wild plants in volumes that can threaten the future of the species. Ginseng — collected and sold for medicinal purposes — is one pertinent example, as are various wildflowers unscrupulously dug from the wild and sold to naïve (or uncaring) gardeners. From a conservation perspective, the best policy is to leave natural communities intact. As the saying goes, “Take nothing but photographs, leave nothing but footprints.”
Common Plant Species
Common plant species are too numerous to discuss individually within the structure of a chapter focused generally on botany. Considered here are some strategies for gaining familiarity with the plants that will be encountered most frequently in Virginia. The diversity of plants in Virginia is sufficiently great that semester-long, college-level courses focused on local flora represent one strategy toward assimilating a working knowledge of our most common plants. Avid naturalists, however, can take advantage of readily available resources and learn much of the same information on their own.
One can learn much by using illustrated guides and matching pictures with plants encountered in the field. The Virginia Department of Forestry publishes a booklet on the “Common Native Trees of Virginia” (see Additional Resources); given the prominence of forests in the natural vegetation of the state, learning to recognize common trees should be a goal for every naturalist. There are numerous published guides to trees, trees and shrubs, wildflowers, and ferns targeted to the motivated amateur. It is important to select guides that are geographically appropriate to Virginia; because many of the most common plants also occur widely throughout Eastern North America, it is generally easy to select an appropriate plant guide.
“Newcomb’s Wildflower Guide” (see Additional Resources) stands apart from many of the popular picture-matching guides in that it introduces the use of keys requiring close examination of flower structure. Although it may be more challenging than some other guides written for amateurs, it does have the advantage of bringing the user closer to the sophistication of formal botanical floras.
Joining the Virginia Native Plant Society, attending local chapter meetings, and participating in society-led field trips is another great way to learn from others who share an interest in the plant life of Virginia.
Rare Species, Rare Communities, and Endemic Species
Rare Habitats in the Eastern Deciduous Forest
Though hard to explain, for many the novelty of rare species holds a certain fascination. Many rare species also are found in uncommon and aesthetically pleasing natural communities. To understand rare species and communities, it is helpful to consider what the landscape may have been like before European settlers arrived in the new world.
Without debating the role of fire and Native Americans’ use of fire, it is reasonably safe to say that Europeans found a nearly unbroken “sea of trees” — especially once they ascended beyond the coastal marshes. The deciduous forest biome stretched across the land, and it would be more than two centuries before they knew what lay beyond. For the most part, a shady, forested environment dominated the landscape. While many differences existed over this immense area, most forest- and shade-loving herbaceous species would have been common.
In contrast, species favoring high light environments would have been much more limited. Open, sunlit conditions occurred in marshes, around ponds, at rock outcrops, along the flood-scour zone of hard-bottom rivers, and where extreme edaphic conditions didn’t allow a full canopy to develop. In short, only the environmental extremes created a break in the forest — too wet, too rocky, too strongly basic, etc., to allow a permanent forest to develop. In this region, such conditions are usually small, patchy, and effectively isolated from one another. They would have to have occurred with sufficient frequency and over a broad enough geographical area to be able to maintain viable populations or develop endemic species.
Shale Barrens
Good examples of a rare habitat from this area are the mid-Appalachian shale barrens. Stretching in a narrow elliptical pattern within the ridge and valley physiographic province, these areas are underlain by Devonian age shales occur.
Where certain combinations of slope aspect and proximity to an undercutting stream exist, relatively barren natural communities occur that are characterized by loose shale fragments covering most of the ground, sparse herbaceous cover, and a thin canopy of low, scraggly, red cedar, Virginia pine, and several hardwood species. The loose shale fragments creep downslope by gravity and by the continuous undercutting of the adjacent stream, creating unfavorable conditions for seed germination and establishment. For the same reasons, these harsh-looking environments are also relatively fragile and easily damaged by hiking on them.
The shale barrens are home to about a dozen endemic species and a number of rare plant species from the Midwest and West. The endemics include three species of clematis (Clematis albicoma, C. viticaulis, and C. coactilis), Kate’s Mountain clover (Trifolium virginicum), shale barren ragwort (Packera antennariifolius), a buckwheat (Eriogonum alleni), an evening primrose (Oenothera argillicola), and the endangered shale barren rockcress (Arabis serotina), to name the best known. Other species, known as long-range disjuncts, occur hundreds of miles from the nearest populations. A few of these are chestnut lipfern (Cheilanthes eatonii), forked spleenwort (Asplenium septentrionale; so far known only from West Virginia), western wallflower (Erysimum capitatum), and bent milkvetch (Astragalus distortus).
As is often the case with extreme environmental conditions, these tracts of land have little or no agricultural or timber value and are, thus, pristine natural communities. Not only are they aesthetically pleasing, they can also give one the sensation of being transported to arid regions a thousand miles or more to the west. The shale barrens have even been described as the “deserts” of the Appalachians; however, research has shown that the endemic plant species do not experience drought stress. In fact, several even flower during July and August, the hottest and driest months of the year!
There are many additional reasons why a species might be rare, and their distributions and habitats may be clues. As naturalists, an understanding of some of these factors and patterns enriches our outdoor experience and provides a fuller appreciation for and a greater commitment to conserving and preserving what remains.
Rare Plants in Common Habitats
Some species seem to be naturally rare. Typically, these species occur over a broad geographical area but are found very infrequently within that region. A good example is swamp pink (Helonias bullata), which ranges from northern Pennsylvania and New Jersey to northern Georgia but it has fewer than about 50 populations. Helonias is a monotypic genus, that is, a genus with only a single species. Because it has no close relatives, it is thought to be an ancient species that once occurred with much greater frequency but has now died out over much of its range — a species on its way to extinction, you could say. Although infrequent, populations can be very large, containing many thousands of individuals. In this sense, it may not seem rare, but even a slight change in hydrology could eliminate entire populations very quickly. If undisturbed, these populations may persist for thousands of years. We do not want to be the agents to cause its demise!
Other rare species seem to be naturally rare and have simply adopted this life history strategy. A number of orchids fit this scenario. The small whorled pogonia (Isotria medeoloides), for example, occurs at widely scattered locations over much of the deciduous forest biome. Its habitat is relatively abundant and doesn’t seem very special. Why should it be so rare? Monitoring and life history studies have not answered this question, but we have learned that the species can persist for years without flowering, and individuals may remain dormant for one to several years, producing no aboveground parts. Individual plants may produce many thousands of tiny seeds, each requiring an association with soil fungi (a mycorrhizal association) for survival. Land-use patterns on a landscape scale make it very difficult to assess the long-term threats for this species.
Marl Marsh Wetlands
Getting back to the idea we began with, many — if not all — rare species in this region are rare because their preferred habitat is rare or has become rare due to man’s impacts on the environment. One of the best examples pertains to species that occur in marl marsh wetlands, mostly in the limestone valleys of Western Virginia. Drainage is impeded by the development of a layer of relatively pure, lime-rich clay (marl) over long periods of time.
These habitats were once common in the region but have been lost or badly degraded as landowners have attempted to reclaim the land for agricultural purposes. Only a few, mostly small, sites remain intact. Many others have persisted in a degraded condition due primarily to the fact that groundwater seepage, springs, and artesian wells were so strong that attempts at drainage have failed. A long list of rare species occurs in these communities: water horsetail (Equisetum fluviatile), tufted hairgrass (Deschampsia caespitosa), sedges (Carex schweinitzii, Carex prairea, Carex suberecta), smooth loosestrife (Lysimachia quadriflora), buckbean (Menyanthes trifoliata), rough avens (Geum laciniatum), and queen of the prairie (Filipendula rubra). Many of these find their primary areas of occurrence north and west of the Appalachians.
Rare Habitats of Southeast Virginia
While there are many other examples we could touch on, we should mention the pine barren and longleaf pine-turkey oak communities of Southeastern Virginia. Though located at the northern end of their natural range in Southeastern Virginia, these communities occupied thousands of acres in presettlement times. They have been eliminated by a combination of the pine tar industry, subsequent conversion to pine plantation for timber production, and fire suppression.
Fire is the natural agent that maintains these communities in a more-or-less perpetual disclimax, i.e., a stalled succession. The elimination of natural fires has led to succession from open pine savanna supporting many sun-loving species to densely vegetated forests where the latter are not able to survive.
Man’s effect on the environment is two-sided in this case. While on one hand we have virtually eliminated a whole segment of our natural heritage, we inadvertently preserved remnants of this flora in power line and pipeline corridors where mowing and spraying provided open, sunny conditions where some of these species have been able to survive.
Richness of Virginia Plant Life
Virginia plant life is rich due primarily to a great diversity of climate, elevation, and substrates creating a wealth of habitats that support common and rare species alike. Over thousands of years, as plant species migrated in response to changes in climate, the unglaciated we now know as Virginia has, in a sense, been collecting elements from the north, south, and west to produce a fascinating array of species and natural communities that continue to be revealed. Much awaits the curious naturalist!
Techniques and Methods for the Study of Plants
Inventories and Checklists
Ecologists and land managers often need descriptive statistics of plant communities. One critical piece of information is the basic inventory or checklist of species, a simple list of every single plant species that occurs within the prescribed boundaries of the study site. Inventories can be assembled through repeated observation of the vegetation, recording the different species as they are encountered. Since some species are ephemeral or highly seasonal in their appearance, especially in the temperate zone, it is often necessary to extend observations through at least one year. One proceeds with observing and compiling until the whole site has been observed multiple times and the rate of encountering species new to the list drops to a negligible level.
For very large study areas, a sampling study may be needed; perusing every nook and cranny of a large area is impractical.
For very large study areas, a sampling strategy may be needed; perusing every nook and cranny of a large area is impractical. The sampling strategy might profitably include examination of each distinctive type of habitat within the large area, and it might include adoption of some of the quantitative procedures, such as quadrats or transects, that are described later in this section. In addition, other sampling procedures are available. Any inventory or checklist should be supported by at least one voucher herbarium specimen for each species included in the list.
Beyond a basic inventory or checklist, it is often useful to have additional information about each of the plant species present in the region. This additional information is often obtained by recording quantitative data following a particular sampling strategy. Some useful quantitative statistics used in vegetation analysis include the following:
- Abundance is an estimation of the numbers of individuals present, often using arbitrarily defined abundance classes, such as rare, occasional, frequent, abundant, and very abundant. The definitions are arbitrary, but “rare” should reflect just a very few encounters in the sample, “very abundant” should apply to individuals that are consistently present and constitute a very high proportion of all individuals in the sample, and “frequent” should reflect a condition in the midrange, with “occasional” and “abundant” as intermediate between the midpoint and the extreme conditions.
- Density, on the other hand, is based on actual counts. The relative density is expressed as a ratio: the number of individuals for each species divided by the number of all individuals in the sample. Density can also be expressed in terms of number of individuals per unit area.
- Cover — like density and abundance — reflects the number of individuals present but also takes into account the effect of plant size because one or two large trees may have far greater impact on a local community than dozens of very small herbs. Cover reflects the effects of the number of individuals and their mass. Cover can be estimated or measured as the surface area of foliage for a given species projected on the ground. If the community under study is stratified (as in a forest) and consists of multiple layers of foliage belonging to plants of different height classes, then cover should be estimated for each stratum or layer of foliage. In other words, measure or estimate the coverage of each species of herb, do the same for each species of shrub, and then do the same for each species of canopy tree. An alternative approach is to base measurement of cover on stem or trunk diameter at the diameter at breast height (DBH), 4.5 feet above the ground. The idea is that canopy and overall mass will be proportional to DBH. DBH-based measures of cover, of course, do not apply to herbs. Cover is often used to express the ecological dominance of a given species of plant.
- Sociability is a measure of how a particular species of plant grows, whether as single isolated individuals or clumped. Most forest trees and many shrubs and herbs occur as isolated individuals. Other sociability categories apply most readily to shrubs and herbs that have a means of asexual or vegetative propagation that gives rise to clumps of various sizes. Some standard categories of sociability include:
- Shoots growing singly.
- Scattered groups of tufts of plants.
- Small, scattered patches or cushions.
- Large patches or broken mats.
- Very large mats or nearly pure populations that carpet the area.
- Importance is a statistic derived from a combination of relative density, relative dominance (cover), and relative frequency. Details for the calculation of importance values and related concepts like the continuum index, persistence, constancy, and fidelity can be found in ecology texts or specialized works on vegetation analysis.
Quantitative methods generally require adoption of a defined sampling method. Two of the most commonly used sampling methods are based on quadrats and transects.
Quadrats/Study Plots
A quadrat or study plot is a defined area within which all the plants are recorded and measured. As the name implies, quadrats are often four-sided geometric figures, either squares or rectangles. The actual shape is less important than adoption of a constant size area from which all samples and data will be taken. Hence, sometimes ecologists will employ circular “quadrats,” largely because the perimeter is easy to define using a center post and a string to circumscribe the boundary of the study plot. Square or rectangular quadrats for forest plots can be defined by corner stakes driven into the ground with string to define the edges. These plots are usually oriented with the edges aligned north-south and east-west via compass to facilitate constructing reasonably accurate rectilinear study plots.
One of the challenges of vegetation analysis is to locate the quadrats in such a way that
the vegetation sampled is representative of the region but also to locate them with sufficient randomness that the investigator does not bias the sampling process. For a study of plant communities dominated by small herbs, preformed frames made of thin pieces of wood or circular hoops of plastic (like a hula hoop) can be tossed randomly into the vegetation to define the quadrats. Quadrat shape is not insignificant. Studies have shown that elongate rectangular quadrats often enclose a more diverse assemblage of plants and, therefore, characterize the overall community more efficiently than square or circular quadrats. The explanation for greater efficiency of rectangular quadrats over less elongate shapes seems to lie in the greater probability that an elongate shape will intercept more microhabitats. Dimensions of the quadrat should be large enough to enclose a statistically significant number of individuals but small enough to make counting all individuals a reasonable undertaking.
Transects
Transect methods involve sampling along a line. End points of the line are fixed, a string is stretched between the two end points, and every plant that is intercepted by the transect is recorded and measured. This technique works well for herbaceous plants because it is easy to superimpose the string-defined transect over the vegetation. It is difficult to run the string directly through tree trunks, so the technique requires some modification for forest applications.
For woody vegetation, one way to use a strictly linear transect would be to characterize the canopy directly over the string, recording the identity and numbers of each canopy species that occurs overhead, and then tallying the distance along the transect that is occupied by each species of canopy tree. Another modification would be to sample every tree that occurs within one meter to the right or left of the transect line. In effect, this belt transect technique amounts to sampling a very long and narrow quadrat, but defining the single-line transect is much quicker than staking out a four-sided quadrat. Use of strings, cables, or ropes that are premarked with standard units of length (by meter, for example) offers obvious advantages during data taking.
One of the advantages of transect-based techniques is that transects can be oriented by design to intercept any sort of environmental gradient so the vegetation data can be directly related to changes in the habitat. For example, species composition may reasonably be expected to vary with distance up and down a slope or from the edge of a body of water to some nearby drier habitat. Transects that cross an ecotone or transition from one ecological community to another provide a good way to contrast the two communities in a quantitative way.
Other Sampling Methods
Other sampling procedures have been developed, such as the variable radius (or variable plot), the loop, and the quarter methods. Details about these techniques can be found in ecology texts or specialized works on vegetation analysis.
Herbarium Specimens
Any serious attempt to document plant life in a defined region requires accurate and verifiable identifications. Herbarium specimens can serve as vouchers for the identifications used in any such study. These are pressed and dried museum specimens that represent each kind of plant studied in the field. It is not always possible to identify species in the field, and the voucher provides a means for identification at a later date. Moreover, identifications made in the field that are not backed by vouchers are little more than assertions made by the ecologist. Names of organisms used in an ecological study that are tied to voucher specimens can always be verified.
Every effort should be made to secure vouchers that contain flowers or fruits or spore-bearing structures that will prove essential in making an accurate determination (identification). The essential link is to include the collection number of each and every voucher species with the ecological data. That collection number can then be traced to an actual herbarium specimen that can be consulted, and the field identification can be verified or refuted. If no voucher is present and some question arises about the true taxonomic identity of a plant included in a study, there are no easy means to remove the cloud of doubt. Vouchers are absolutely essential for critical ecological work.
Making herbarium specimens starts with taking data in a field notebook. It is usually convenient to start with date and locality information. Locality information is usually recorded sequentially from the most inclusive geographic category to the smallest or most specific. Hence, one might start with the state and county and proceed to site-specific details. Given the widespread availability of GPS systems, latitude and longitude should also be recorded. Each specimen should receive a unique field number; duplicate (or multiple) specimens of the same plant taken at the same location on the same date should all receive the same field number.
Following locality data, it is often useful to note ecological observations, nature of habitat, soils, exposure, associated vegetation, etc. If anything is known about the plant’s identity, the name or group to which it belongs should be noted next to the field number. Finally, the collector should record any aspect of the plant that will not be preserved in the pressed and dried specimen. For trees, it is standard practice to record height and DBH; as needed, it is good practice to record flower and fruit colors, fragrances, presence of colored sap or latex, and any pollinators observed visiting the flowers.
Once the field notes are complete, the specimen should be collected. Every effort should be made to make the collection as complete as possible, including roots for herbaceous plants, as well as flowers and/or fruits and seeds (or spore-bearing organs for ferns and fern allies). The amount taken should be sufficiently generous to allow identification of the pressed and mounted specimen. HOWEVER, one must always be sensitive to conservation issues when collecting specimens; plants listed as rare or threatened or any plant present in local populations consisting of less than 20 individuals should be documented by nondestructive means, such as photography. Often, collections are held in plastic bags (or more traditionally, in a metallic box or vasculum) until they can be pressed and dried.
A standard plant press consists of two sturdy wooden frames and a series of cardboard corrugates, blotting papers, and sheets of folded newsprint. Folded newsprint may be a little smaller, but the other elements of a standard plant press are 12 inches by 18 inches. The plant is inserted inside the folded newsprint that is then sandwiched between blotters and cardboard. The press is built in successive repeating layers of newsprint-blotter-corrugate, with the press frames at the top and bottom of the stack.
Care should be taken while pressing to arrange the plant to maximum advantage, so both leaf surfaces (upper and lower), flowers, and fruits (or spores) are well-exposed. When all plants have been inserted into the press, the frames are strapped down securely and the press is exposed to warm, dry air until the plants are warm and dry to the touch. Specimen labels are prepared from the field notes and collated with the proper specimens that are then deposited with an herbarium curator for study, identification, and storage.
Photography
Photography is an important adjunct to the study of plant diversity and its documentation in ecological settings. Whether film or digital cameras are used, a few basic principles will help in the production of good quality photographs of plants.
- Lens and camera quality are important. Many consumer-level cameras designed for candid snapshots of people lack the ability to resolve sufficient detail or focus for close-ups (or both) and so are of limited utility in serious plant photography. Specially defined “macro” lenses are excellent for close-ups, but good results can be obtained from other devices, like attachment lenses (in several degrees of “+” magnification) or lens extension tubes.
Good lenses, however, cannot compensate for bad lighting. Generally, for detailed close-up work, bright but flat (low-contrast) light is best. Bright overcast conditions are generally better than direct, full sunlight. Use of reflectors to increase light intensity or to fill in shadows cast by high-contrast light sources is very beneficial. - Camera stability is also critical. Any movement of the camera during exposure will result in blurred images. As light intensity decreases, both exposure (open shutter) time and the need for rock-solid stability increase. Tripods (or monopods) become indispensable for exposure times less than 1/60th of a second.
- Finally, one of the easiest ways to take better photographs is to take a lot of them and be particular about which ones you keep or use. You may need only one image, but if you take 20 shots, you have the luxury of selecting the best of 20.
Sampling Algae
Although technically not plants (as defined in this chapter), there are special techniques for sampling and studying algae — simple photosynthetic organisms that inhabit aquatic habitats. Algae that occur in the water column can be sampled most conveniently by plankton nets that are tossed in the water and pulled to shore via a cord or towed behind a boat. Net mesh dimensions should be appropriate to the size of the algae present.
Algae caught within the net are then studied by compound microscope for identification. The different kinds of algae present in the sample can be quantified as long as care is taken to account for the volume of lake or pond water that passed through the net when the sample was taken, the volume of the drop placed on the microscope slide, and the portion of the drop actually used to identify and count each species of algae. Details can be found in aquatic ecology or phycology textbooks.
BioBlitz
A popular way to highlight biodiversity in a defined geographic area is to conduct a BioBlitz — a concerted effort by a team of specialists to locate and identify all the living organisms in a study area. Usually there is a defined time limit to the event, often a single 24-hour period. Botanists who participate in BioBlitz events often focus on particular groups of plants. Many botanists have wide expertise with the vascular plants, but specialists are often required to inventory bryophytes (mosses and liverworts), lichens, or fungi with reasonable accuracy. Sampling techniques (quadrats and transects) and herbarium specimens are often made as part of the effort. In addition to much camaraderie, the end product of a BioBlitz is a detailed inventory of all organisms found in the study area.
Study and Review Questions
- What are the major taxonomic groups of plants found in Virginia? Which of these groups is, by far, both the most abundant and diverse? What environmental factors are important in determining the geographic distribution of plants in Virginia?
- What are the major roles of plants in the flow of food and energy through the ecosystem? In what ways do plants both form and define distinctive ecological communities?
- In what fundamental ways does the basic biology of plants and animals differ? How is their basic biology similar?
- What are some of the noteworthy adaptations of plants to dry habitats? To wet habitats? What aspects of flower structural diversity can be interpreted as adaptations to different modes of pollination? What different adaptations for seed dispersal can be found in plants?
- Why have classifications of plants changed over the centuries? What sorts of evidence form the basis of modern plant classification?
- Of the diverse aspects of stem morphology found in different plants, which are useful in providing insights to identification? For leaves? For flowers? For fruits?
- What are the most urgent threats to the plant life of Virginia?
- What are some of the most abundant and diverse families of plants found in Virginia? What characters can be used to recognize these families?
- While many plants are common and abundant, for those that are not, what explains their rarity? Do rare plants always occur in rare or uncommon habitats? What is an endemic species?
- How do botanists compile inventories of plant species for a given geographic area?
- What aspects of plant community structure can be elucidated by transect- and quadrat-based measurements?
Acknowledgments
Ruth Douglas assisted in planning and outlining topics for inclusion in the botany chapter. Thomas Wieboldt contributed to the sections on plant distributions, rare species, rare communities, and endemic species.
Sources of Illustrations
Britton, N. L., and A. Brown. 1913. An Illustrated Flora of the Northern United States, Canada and the British Possessions, 2nd ed. 3 vols. New York: Charles Scribner’s Sons.
Goebel, K. (1900) 1905. Organography of Plants: Especially of the Archegoniatae and Spermaphyta. 2 vols. English edition by I. B. Balfour. Oxford: Clarendon Press. [Available as a 1969 reprint by Hafner Press, New York.]
Gray, A. 1868. Gray’s School and Field Book of Botany. New York: Ivison, Blakeman, Taylor & Co.
Grout, A. J. 1903 Mosses With Hand-Lens and Microscope New York: published by the author.
USDA (U.S. Department of Agriculture). National Resources Conservation Service. “PLANTS Database.” http://plants.usda.gov/index.html.
Wood, A. (1870) 1889. The New American Botanist and Florist, rev. ed. Revised and edited by Oliver R. Willis. New York: A. S. Barnes and Company.
Additional Resources
Fleming, Gary P. and Karen D. Patterson 2017. Natural Communities of Virginia: Ecological Groups and Community Types. Natural Heritage Technical Report 17-07. Virginia Department of Conservation and Recreation, Division of Natural Heritage, Richmond, Va. www.dcr.virginia.gov/natural-heritage/natural-communities/document/comlist04_17.pdf.
Kent, M. 1992. Vegetation Description and Analysis: A Practical Approach. Boca Raton: Fla.: CRC Press.
Newcomb, L. 1977. Newcomb’s Wildflower Guide. New York: Little, Brown and Company.
Raven, P. H., R. F. Evert, and S. E. Eichorn. 2005. Biology of Plants, 7th ed. San Francisco: W. H. Freeman.
Stern, K. R., J. E. Bidlick, and S. Jansky. 2008. Introductory Plant Biology, 11th ed. Boston: McGraw-Hill.
Townsend, J. 2019. Natural Heritage Resources of Virginia: Rare Plants. Natural Heritage Technical Report 19-15. Virginia Department Of Conservation and Recreation, Division of Natural Heritage. www.dcr.virginia.gov/natural-heritage/document/plantlist19.pdf.
Virginia Botanical Associates. 2011. “Digital Atlas of the Virginia Flora.” www.vaplantatlas.org/.
Virginia Department of Conservation and Recreation. Division of Natural Heritage. 2009. Invasive Alien Plant Species of Virginia. www.dcr.virginia.gov/natural-heritage/invspinfo.shtml.
Virginia Department of Forestry. 2016. “Common Native Trees of Virginia.” www.dof.virginia.gov/infopubs/Native-Tree-ID_2016_pub.pdf.
Weakley, A. S., J. C. Ludwig, and J. F. Townsend, 2012. Flora of Virginia, Fort Worth: BRIT Press. Also see the mobile app at floraofvirginia.org.
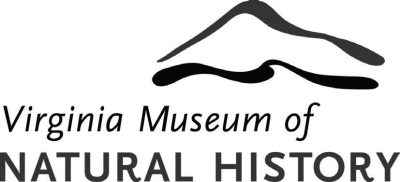

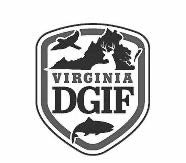
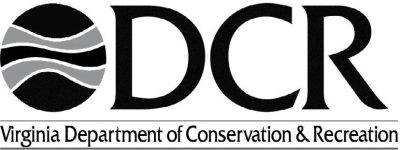

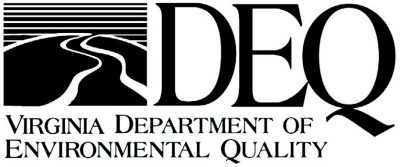
Virginia Cooperative Extension materials are available for public use, reprint, or citation without further permission, provided the use includes credit to the author and to Virginia Cooperative Extension, Virginia Tech, and Virginia State University.
Virginia Cooperative Extension is a partnership of Virginia Tech, Virginia State University, the U.S. Department of Agriculture, and local governments. Its programs and employment are open to all, regardless of age, color, disability, sex (including pregnancy), gender, gender identity, gender expression, national origin, political affiliation, race, religion, sexual orientation, genetic information, military status, or any other basis protected by law
Publication Date
March 22, 2023